Introduction
Literature Review
Alzheimer’s disease (AD) is the most prevalent neurodegenerative disorder associated with aging (Niedowicz, Nelson and Murphy, 2011) and its incidence is expected to grow intensively such that by 2050 over 100 million people worldwide will have developed the dementia (Prince, Bryce and Ferri, 2011). AD is characterized by the progressive loss of cognitive functions, specially, the memory. However, besides compromising the memory, this pathology is responsible for deficits in attention, orientation, organization, in the capacity of planning and judging, among other cognitive functions (Weintraub et al., 2009), leading to the inability of living independently.
Pathological hallmarks of AD are dystrophic neuritis associated with plaques and neurofibrillary tangles within nerve cell bodies in the basal forebrain and neocortical cholinergic pathways (Wszolek, 2008). The widespread neurons loss and synaptic connectivity in AD seem to be caused by the accumulation of toxic species of the β-amyloid (Aβ) peptide (Chen, & Blurton-Jones, 2012). Pharmacological treatments for this disorder are palliative, having the property of slowing down the speed of the disease’s progression. Thus, stem cells-based therapy constitute promising prospects for the treatment of central nervous system (CNS) disorders, once it is known that stem cells (SCs) have a great potential to develop into many types and subtypes of cells, besides being able of in vitro long term survival and self-renewal (Ibarretxe et al., 2012).
Mesenchymal stem cells (MSCs) have important advantages over embryonic SCs, such as the facility for isolation, the capacity to propagate in culture and the property of non-immunogenic characteristics (Bydlowski, Debes, Maselli and Janz, 2009) besides expressing some common antigens in rats and humans (Foudah et al., 2012). Animal models represent a suitable model for in vivo and in vitro studies since there are physiological characteristics similar to those of human (Bugos, Bhide and Zilka, 2009).
SCs-based therapies may effectively support activation of neurogenesis, as well as migration, maturation and integration of newly generated neurons in a functional network through the production of diffusible trophic factors (Okano, Sakaguchi, Ohki, Suzuki and Sawamoto, 2007), enlightening the possibility of SCs to treat neurodegenerative diseases.
Research Question
According to the potential therapeutic application of MSCs for neurodegenerative diseases, the aim of this study was to investigate the effects of SCs grafts on the behavior of rats in an AD model. Our working hypothesis was that engrafted animals would improve their behavior when compared to the non-engrafted group. For the study we used SCs from human Dental Pulp of Deciduous Teeth (DPDTh-SCs), human Adipose Tissue (ATh-SCs) and mice Renal Tissue (RTm-SCs).
Research Design and Methodology
Animals
Experiments were performed in 60 male Wistar rats (300-350 grams in the beginning of the study) from the Animal Breeding Unit of Universidade Federal de Ciências da Saúde de Porto Alegre (UFCSPA). They were housed in groups of three per cage, receiving water and food ad libitum, maintained in a room with a controlled temperature at 24ºC and a 12-hour light/dark cycle (lights on at 7 am). Rats were manipulated according to international laws for ethical care and use of laboratory animals (Guide for Care and Use of Experimental Animals, by the National Institutes of Health, 1996) and experiments were approved by the local Ethics and Research Committee (UFCSPA, protocol no.079-11, project no. 051-11).
Rats were distributed into 5 different groups (12 animals per group): control, AMPA, DPDTh-SCs, ATh-SCs and RTm-SCs. The goal of the first surgery was to lesion the nucleus basalis magnocellular (NBM), an analogous structure to the nucleus basalis of Meynert in humans (nbM). Except for the control group that received saline infusions, all of the others received infusions of AMPA. In the second surgery, except for the control and the AMPA groups which received saline infusions, SCs were implanted. The groups were distributed according to the following:
Group 1: saline + saline
Group 2: AMPA + saline
Group 3: AMPA + DPDTh-SCs
Group 4: AMPA + ATh-SCs
Group 5: AMPA + RTm-SCs
Experimental Procedures
All lesion and graft surgeries followed the same anesthetic and post operative care procedures. For sham surgery, animals underwent the same procedure for lesioning and grafting and received the respect vehicle injection at the same coordinates.
Anesthesia:
Rats were weighed and intraperitoneal (ip) anesthetized with xylazine (10 mg/kg) and ketamine (100 mg/kg).
Surgery for Lesions and Grafts:
Animals were mounted on a stereotactic frame (Insight, Brazil) and a midline incision was made with a scalpel. Bregma was located and the coordinates calculated. Appropriate holes were made through the skull using a dental drill, exposing the dura mater but not touching it. For lesioning, a cannula attached to a 5µL Hamilton syringe was used. Unilateral injections of 0.4µL of AMPA (S-AMPA: Tocris Neuramin, UK) in a 0.05mM sterilized phosphate buffered saline (PH 7.4) were infused at a flow rate of 0.2 µL/minute. Anterior/posterior (AP) coordinates taken from bregma, lateral (L) midline and ventral (V) depth from dura mater, used the following coordinates: rostral AP: +1.0; L: +2.6; V: -7.5 and caudal AP: +0.2; L: +3.2; V: -7.0.
For grafting, an interval of 21 days after lesion surgeries was used as a standard operating procedure. Each lesioned animal received two infusions in the frontal and parietal cortices of 2,5uL of a suspension containing 50.000 cells/uL performing about 125,000 cells per infusion. A total of 250,000 cells was implanted. The infusions were made through a 10µL Hamilton syringe with flap tip removable needle fixed on the measurement arm of the stereotactic apparatus. Each injection took 2.5 minutes (speed rate of 1µL/minute), using the following coordinates for frontal and parietal cortices, respectively: AP: +3,2; L: +3,5; V:-3,0 and AP: -1,0; L: +5,0; V: -2,2.
Cell Preparation Procedure:
The stem cells used in this study were isolated using previously published standard protocols. All cell types were cultivated in Dulbecco’s Modified Eagle’s Medium (Sigma Chemical, St Louis, USA) supplemented with 10% fetal bovine serum (FBS) and 1% penicillin/streptomycin (Gibco BRL, NY, USA). The procedures for isolation of mesenchymal stem cells from different sources are described below. Briefly, adipose tissue was removed from patients submitted to liposuction at Hospital Santa Clara – Complexo Santa Casa de Misericórdia de Porto Alegre (with signed Patients Consentient Term). This liposuction product was washed and submitted to enzymatic treatment with collagenase Type I 0,075% at 37°C for 30 min. The collagenase was neutralized with Dulbecco’s Modified Eagle’s Medium (Sigma Chemical, St Louis, USA) supplemented with 10% fetal bovine serum (FBS). The suspension was centrifuged at 800xg for 10 minutes and cultured in tissue culture flasks. Dental pulp tissue was removed after signed Parent Consentient Term from 30 different patients, aged from 6 to 12 years. From all the teeth, 21 were in advanced levels of resorption and the remaining nine teeth did not show any visible resorption. Pulp tissue was collagenase digested, and the suspension seeded onto 12-well plates. Mice kidney-derived mesenchymal stem cells were isolated by digestion of half kidney with 1 mg/ml of collagenase type 1 in DMEM for 45 min at 37º C followed by pippeting for tissue dissociation. After collagenase neutralization cells were centrifuged at 300xg for 10 min and cells were plated in a well of a 6-well plate.
The non-adherent cells were removed with media changes and subsequent changes made every 3-4 days until cultures reach confluence.
After confluence, the primary cultures were washed in PBS without Ca2+ and Mg2+ (Sigma) followed by incubation for 5 min with 0,25% tripsin-EDTA solution at 37ºC. Passages were done when cultures achieved at least 90% of confluence.
The phenotype of the human cells (n = 5) was analyzed on fifth and tenth passages by flow cytometry for clusters of differentiation (CD)29/PE, CD34/PE, CD44/FITC, CD45/FITC, CD90/FITC, CD117/PE, CD133/PE, CD146/FITC, CD184/PE, Stromal Cell Surface Marker 1 (STRO-1)/FITC and human leukocyte antigen major histocompatibility complex class II surface receptor (HLA-DR)/FITC. On the same passages, cells were differentiated into adipocytes, osteoblasts, and chondrocytes.
Post-Operative Care Procedure:
After surgeries, animals were caged individually and given wet mashed food until normal drinking and feeding was returned. The cage’s floor was covered with white crepe paper to facilitate checking food intake, defecation and urine conditions. Wet and dry pellets were placed in the cages to monitor food consumption. Besides this, twisting, rolling over, shaking and convulsive fits were observed as effects of the lesion. Animals were considered recovered when the pre-surgery weight was re-gained, there was no blood in the urine and normal movement was re-established. They were then group-housed and daily monitored.
Behavioral Tests
After 21 days of the surgical procedures, experiments were carried out using the following sequence of testing: open field, elevated T-maze and object recognition. At the end of each test, animals were given a three-day interval. Tests run in the order listed, from least invasive to most invasive.
Open Field (OF) was used to investigate the influence of lesions and grafts on motor activity and exploratory behavior of the subjects. Animals were exposed to the OF for 2 minutes, 4 days in a row. The whole procedure was recorded. The number of crossings and the avoidance of the central area were taken under consideration. According to Bogdanov (2013), a decrease in the ambulation may be used to investigate anxiety and fear, and the avoidance of the central area may indicate a restraint of dangerous environments.
The OF consisted of a circular wooden arena (80cm in diameter with walls 40cm high). The floor was divided into 12 segments of approximately the same area (Open Field — Rats. Insight, Brazil). Animals were taken to a pre-test waiting room for 30 minutes before the experiment started. The test sessions were carried out in the presence of a researcher and they were recorded by a video camera placed 1,5m above the apparatus. Rats were carefully handled and placed individually at the centre of the apparatus, and after 2 minutes of testing they were placed back in the group cage.
Elevated T – Maze (ETM) was used in the study to evaluate two types of fear responses: learned and innate. Repeatedly placing the rat inside the enclosed arm to explore the maze allows the animal to learn not to enter open arms (inhibitory avoidance learning). Re-exposing the animal to the situation after a time interval assesses memory of emotional related behavior, whereas placing the animal in an open arm produces unlearned escape responses into the enclosed arms (Conde et al., 2000).
The apparatus consisted of an enclosed perpendicularly positioned arm at a right angle to two open arms elevated 50cm from the ground (Elevated T-Maze — Rats. Insight, Brazil). Placing the animals repeatedly into the enclosed arm allows them to explore the maze and learn inhibitory avoidance of the open arms. Placing them at the end of one open arm produces an escape response generally to the enclosed arm (Zangrossi and Graeff, 1997). Re-exposing the animals to the same situation after a time interval assesses memory of these emotionally related behaviors (Conde et al., 2000).
Conde and colleagues (2000) demonstrated that a multi-trial training-to-criterion (criterion of 5 minutes not evading to the open arms) increased avoidance and escape performance on later trials. The following behaviors were scored in the ETM: number of trials before reaching the criterion of 300 seconds in the enclosed arm (acquisition), latency to return to the enclosed arm after the criterion was obtained (escape 1), and latency to go to the enclosed arm (escape 2).
Object Recognition (OR) was carried out in the same arena used in the OF (Insight, Brazil). All animals were habituated to the environment 30 minutes before the sessions. The two objects were made out of metal and plastic and were fixed to the arena’s floor with adhesive ribbon. The training session was held 24hours before the trial, and the animals were placed in the arena containing one object, being left to freely explore it for 15 minutes. The test was repeated 24 hours later to evaluate long-term memory, following the protocol described by Ferreira (2009). In the trial, a novel object was put along with the familiar and the animal was left to explore both objects freely. Exploration was recorded. Time spent to explore the objects was analyzed by a blind observer.
Perfusion and Fixation
At the end of the behavioral testing, animals were anesthetized with xylazine (10 mg/kg ip) and ketamine (100 mg/kg ip) and transcardially perfused with 300mL of heparanized saline followed by 4% paraformaldehyde (PFA) in a 0.1M sodium phosphate buffer saline (pH 7.4). The heparanized saline was cooled and PFA was at room temperature. After pre-wash and fixation perfusions, brains were removed from the skull, post fixed for 24hours at 4ºC in PFA and transferred to 30% sucrose in PBS solution cryoprotection. Brains were kept in sucrose for at least 10 days and then cut at 40 micrometers in a cryotome.
Immunostaining
Immunostochemical methods were used to identify lesion sites, phenotypes of grafted cells, migration and reaction of the host tissue to the implanted material. Frozen sections were washed in PBS 3 times for 5 minutes. Primary antibodies were diluted in anti-NeuN, anti-hNuclei and anti-ChAt and put in the shaker for 24hours. Frozen sections were then washed in PBS 3 times for 5 minutes and the secondary staining used were goat anti-mouse, goat anti-rabbit and donkey anti-goat (depending on the primary used) Alexa 488 and 568 (Molecular Probes, UK), green and red, respectively, at concentrations of 1:400. Hoechst 33342 (Molecular Probes) counterstaining was used in all studies. Hoechst is a cell-permeat nuclear dye that emits blue fluorescence at UV light when bound to DNA (double-stranded desoxyribonucleic acid). The images taken from treated sections were analyzed in a fluorescent microscope (Olympus, BX 51).
Statistical Analyses
Data from OF and OR were expressed as mean ± SD and standard error (SE). The intergroup data were analyzed using one way ANOVA, followed by the Bonferroni post hoc test. Data from ETM were analyzed using Kruskal-Wallis. Nonparametric post hoc comparisons were analyzed by U Mann-Whitney test followed by Bonferroni’s correction. A ‘p’ ≤ 0.05 was considered statistically significant for all tests and data analyses were held in the SPSS 17 package for Windows.
Results
Open Field. In the first day of the OF, there was statistically significant difference among groups on the crossing variable (p=0,001), as shown in Table 1. The Bonferroni post hoc test showed significant difference between the Control group and ATh-SC group; the AMPA group and RTm-SCs group; and the RTm-SCs and ATh-SCs implanted groups. In the second day of testing it was possible to verify statistical difference among 3 variables: time spent in the periphery area (p= 0,012), time spent in the center area (p= 0,012) and latency (p= 0,012). Bonferroni’s post hoc test demonstrated powerful differences between the AMPA group and control group; the AMPA group and DPDTh-SCs group; and the AMPA group and ATh-SCs group (see Table 1). There was no other significant statistical value in the testing days 3 and 4.
Table1: Open Field: Significant Variables from First and Second Days
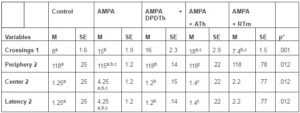
M = media. SE = standard error.
Periphery 2 = time spent in the periphery area in the second day.
Center 2 = time spent in the center area in the second day.
Latency 2 = time spent to leave the center area in the second day.
Control = Saline + Saline (n=8), AMPA = AMPA + Saline (n= 8), AMPA + DPDTh = AMPA + SCs implant from human dental pulp from deciduous teeth (n= 9), AMPA + ATh = AMPA + SCs implant from human adipose tissue (n= 10), AMPA + RTm = AMPA+ SCs implant from mice renal tissue (n= 9).
p* value considered < 0,05. One way ANOVA, Bonferroni post-hoc test: significance is expressed among the groups by the identical letters “a”, “b” and “c”.
Crossings (see Figure 1) decreased along the testing days in two of the groups: AMPA and ATh-SCs group. The other groups increased their crossings, but no statistical values were shown.
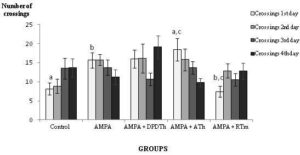
Figure 1: Open Field: Number of Crossings
Control = Saline + Saline (n=8), AMPA = AMPA + Saline (n= 8), AMPA + DPDTh = AMPA + SCs implant from human dental pulp from deciduous teeth (n= 9), AMPA + ATh = AMPA + SCs implant from human adipose tissue (n= 10), AMPA + RTm = AMPA+ SCs implant from mice renal tissue (n= 9). p* value = value used p < 0,05. One way ANOVA, Bonferroni post hoc test: significance is expressed among the groups by the identical letters “a”, “b” and “c”.
Elevated T – Maze. Kruskal-Wallis’ test showed statistical difference in the ‘latency’ variable from the second testing day (p=0,028). The U Mann-Whitney’s test followed by the Bonferroni’s correction demonstrated differences between the AMPA group and the ATh-SCs group (p = 0,005); and between the control group and ATh-SCs group (p=0,005) (see Figure 2). No other statistical difference was pointed out in this behavioral test (see Table 2).
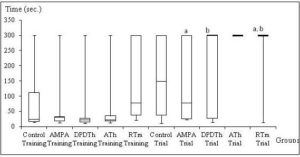
Figure 2: Elevated T- Maze: Inhibitory Avoidance from Training and Trial
Control = Saline + Saline (n=8), AMPA = AMPA + Saline (n= 8), AMPA + DPDTh = AMPA + SCs implant from human dental pulp from deciduous teeth (n= 9), AMPA + ATh = AMPA + SCs implant from human adipose tissue (n= 10), AMPA + RTm = AMPA+ SCs implant from mice renal tissue (n= 9). p* value = value used for a ‘p’ < 0,05. Kruskal-Wallis’ and Mann-Whitney’s Tests: significance is expressed among the groups by the identical letters “a”, “b” and “c”.
Table 2: Elevated T-Maze
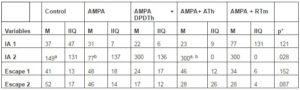
M = median, IIQ = interquartil interval, IA= inhibitory avoidance, Control = Saline + Saline (n=8), AMPA = AMPA + Saline (n= 8), AMPA + DPDTh = AMPA + SCs implant from human dental pulp from deciduous teeth (n= 9), AMPA + ATh = AMPA + SCs implant from human adipose tissue (n= 10), AMPA + RTm = AMPA+ SCs implant from mice renal tissue (n= 9). Number 1 represent the training and number 2 represent the trials. p* value = value used for a ‘p’ < 0,05. Kruskal-Wallis’ and Mann-Whitney’s Tests: significance is expressed among the groups by the identical letters “a”, “b” and “c”.
Object Recognition. There was no statistical significance in the intergroup comparison between the variables ‘time spent exploring the novel object’ and ‘time spent exploring the familiar object’ (Table 3). However, there was statistical significance among the intergroup medias in the analyses of the ‘difference between exploration of the novel object and the familiar object’ (p = 0,03). Bonferroni’s post hoc test identified a powerful difference between the control group and ATh-SCs group (p= 0,03).
Table 3: Object Recognition
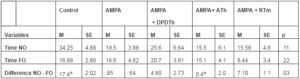
M = media, SE = standard error, NO = novel object, FO = familiar object, Control = Saline + Saline (n=8), AMPA = AMPA + Saline (n= 8), AMPA + DPDTh = AMPA + SCs implant from human dental pulp from deciduous teeth (n= 9), AMPA + ATh = AMPA + SCs implant from human adipose tissue (n= 10), AMPA + RTm = AMPA+ SCs implant from mice renal tissue (n= 9). p* value = value used p < 0,05. One way ANOVA, Bonferroni post hoc test: significance expressed with identical letter "a".
Histology
It was possible to identify, in all AMPA-infused animals, smaller or none cholinergic expression in the ipsi-lateral side of the lesion. Also, in the NBM examination it was observed cholinergic stained cells in the contra-lateral side of infusion, implying successful cholinergic depletion (Figure 3).
Human and mice implanted stem cells were not positively visualized in the immunohistology examination. The anti-Human-Nuclei antibody was previously tested and the process showed positive human cells in a control tissue. However, previous studies have shown functional recovery without positively recognizable implanted human cells presence after a period of time (Oliveira, 2003; Smith et al. 2011; Katare, et al. 2014).
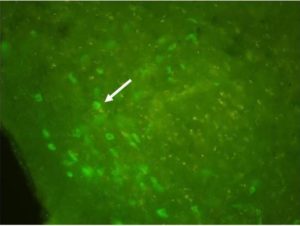
Figure 3: Choline Acetiltransferase-Positive Cells Expressed in the Contralateral NBM of AMPA Infused Animals. The White Arrow Indicates a Neuronal Cells Expressing Cholinergic Phenotype. There Were No Detectable Cells in the Opposite Site
Discussion
In the OF, according to Harsha and Anilakumar (2013), an increase in crossings along the testing days could be explained by the animal’s acquaintance to the environment, suggesting the idea of safeness to exploring the apparatus. Our results show that the animals in Control group, DPDTh-SCs and RTm-SCs groups behaved in a similar way, exploring more the apparatus along the testing days. These results may suggest that the grafted animals turned to a similar behavior as the Control group and this could be result of a easier familiarization with the novelty of OF environment.
The differences observed in the first day of crossings between RTm-SCs group and the AMPA group, may suggest the implants increased the exploratory behavior in implanted rats. Unlike the animals RTm-SCs implanted, the AMPA group, spent more time exploring the center area of the apparatus, reflecting a riskier behavior.
In the ETM, apparently the three implanted groups showed a better performance than the AMPA group, but only one of them exhibited statistical meaning. In the trial, it was identified statistical significance between ATh-SCs group and the AMPA group in the ‘acquisition of inhibitory avoidance’. Perhaps it only happened in the ATh-SCs group because the test was not specific to evaluate the ability of learning new tasks or because of the small number of animals in the groups.
It was not possible to identify statistical differences between the control group and the AMPA group in the OR test. Yet, it was interesting to notice a better performance of the ATh-SCs group than the control group. More studies should be done to understand the reason of this finding. The lack of significant differences in this behavioral test may also be explained by the low specificity of the test to evaluate long-term memory, as well as the small number of animals per group.
There is a number of articles showing in vivo studies for different experimental models involving MSCs from several species, suggesting that MSCs may have function across species barriers (Li, Ezzeralab and Cooper, 2012). Human MSCs have been tested in at least seven different recipient species, and MSCs from four different species were demonstrated to function across species barriers (Li et al., 2012). Therefore, it would not represent a reason this could be the reason of such improvement in the animal’s behavior.
It was not possible to identify the implanted cells in the histology. Some studies have shown functional effects in different models and instances after human implanted stem cells but no histological positive cells. The possible reason is that the cells were dead or depleted by immunoreactions. Few studies show short period of cell survival and still functional recovery (Smith, et al., 2011). None the less, the migration of the SCs to injured tissues in animal models is associated to not yet clearly understood cell mechanisms. Such mechanisms are likely to be involved in the production of paracrine, anti-inflammatory and anti-apoptotic factors (Roux et al., 2011). Paracrine effect is defined as a form of communication between two different cells. One releases chemical mediators to its immediate environment, resulting in a change in the behavior of a cell in its adjacent environment (Anthony and Shiels, 2013).
In a study of organ bio-distributions of transplanted MSCs in mice with acute kidney injury, Cheng and colleagues (2013) found out that after intravenous administration, MSCs were largely localized in pulmonary capillaries but cells survived only transiently. Transplanted MSCs did not migrate to kidneys from peritoneal cavity nor renal subcapsular space, but it was identified the presence of over forty regulatory cytokines protecting renal tubular cells from cisplatinum-induced cytotoxicity.
Besides, Joyce and colleagues (2010), in a study of acute cerebral injury, demonstrated that a very limited number of grafted SCs characterized a stable implant; and SCs revealed an intense migration to injured sites, promoting endogen neuronal growth, decreasing the apoptosis or inflammation. However, in a transient way, once they had disappeared in time (Joyce et al., 2010).
Study Limitations
Even though there were differences in behavioral performance, the histology was not conclusive for cell identification and implanted cells were not traced. Human nuclei antibody was used to detect human implanted cells and, although it was effective in a control human tissue, the implanted cells were not detected. However, the immunohistology was conclusive to detect lesion in AMPA-infused animals.
The use of AMPA to produce cholinergic lesions of the basal forebrain has been reported in several studies. These lesions mimic some histological and behavioral characteristics of AD such as loss of cortical ChAT expression and memory decline. Despite of that, the relationship between cortical cholinergic deafferentation and associated cognitive deficits has been questioned. The reason is that focal injections of AMPA targeting cholinergic neurons in the nucleus basalis magnocelular produced great cholinergic depletion but less severe learning, retention and attentional deficits (Page et al., 1991; Oliveira, 2003).
However, other studies revealed that unilateral lesions of the nucleus basalis magnocelular produced substantial deficits in sensorimotor and spatial learning (Abdula et al., 1994). Therefore, unilateral lesions produced greater cognitive disturbance than bilateral lesions possibly due to sensorimotor deficits produced by the unilateral cholinergic depletion.
More exams need to be done to identify immune activation markers such as CD11b, CD4 and CD40, as well as markers for mice cells. Besides this, more specific behavioral test should be done, such as the water maze or passive avoidance tests.
Conclusions
Xenografts have shown promise in the treatment of neurological diseases. Implants from SCs demonstrated an improvement in the performance of groups in a model for AD in some of the behavioral tests. The existence of behavioral improvement is not only associated exclusively to implants survival. Rehabilitation of tissues may occur due to innate mechanisms of the SCs to migrate to the lesion site, in particular, inflammations, apoptosis or hypoxia, releasing trophic factors that accelerate the endogen repair (Joyce et al., 2010).
Aside from the observation that SCs infusion may induce autologus release of a multitude of growth factors and cytokines to induce the reparative process, SCs release microvesicles and exosomes that may produce paracrine factors required for tissue repair (Anthony and Shiels, 2013). Paracrine factors released by transplanted cells accounted for improvements, and it appears that the route of cell transplantation is not critical for realizing benefits of cell therapy (Cheng et al., 2013). Paracrine-mediated tissue repair is to be exploited via genetic engineering of stem, progenitor or other cells to deliver various beneficial mediators to stimulate regenerative processes in damaged tissues (Anthony and Shiels, 2013).
Stem cell—based therapy represents an emerging therapeutic approach by which incurable diseases may be treated (Marchetti, Krohne, Friedlander and Friedlander, 2010). Although more should be known to clarify all the mechanisms of cellular paracrine effects in the host’s tissues, there is a good perspective that cell therapy may be used to treat neurodegenerative diseases.
Acknowledgement
Grants from Brazilian Agencies: Conselho Nacional de Desenvolvimento Científico e Tecnológico (CNPq) andCoordenação de Aperfeiçoamento de Pessoal de Nível Superior (CAPES).
(adsbygoogle = window.adsbygoogle || []).push({});
References
Anthony, D. F. & Shiels, P. G. (2013). “Exploiting Paracrine Mechanisms of Tissue Regeneration to Repair Damaged Organs,” Transplantation Research, 2 (10).
Publisher – Google Scholar
Bernardi, L., Luisi, S. B., Fernandes, R., Dalberto, T. P., Valentim, L., Chies, J. A. B., Fossati, A. C. & Pranke, P. H. L. (2011). “The Isolation of Stem Cells from Human Deciduous Teeth Pulp is Related to the Physiological Process of Resorption,” Journal of Endodontics, V. 37, P. 973-979.
Publisher – Google Scholar
Bogdanov, V. B., Bogdanova, O. V., Koulchitsky, S. V., Chauvel, V., Multon, S., Makarchuk, M. Y., Brennan, K. C., Renshaw, P. F. & Schoenen, J. (2013). “Behavior in the Open Field Predicts the Number of KCI-Induced Cortical Spreading Depressions in Rats,” Behavioural Brain Research, 236 (1), 90-93.
Publisher – Google Scholar
Bugos, O., Bhide, M. & Zilka, N. (2009). “Beyond the Rat Models of Human Neurodegenerative Disorders,” Cellular and Molecular Neurobiology, 29 (6-7), 859—869.
Publisher – Google Scholar
Bydlowski, S. P., Debes, A. A., Maselli, L. M. F. & Janz, F. L. (2009). “Características Biológicas Das Células-Tronco Mesenquimais,” Revista Brasileira de Hematologia e Hemoterapia, 31 (Supl.1), 25-35.
Publisher – Google Scholar
Chen, W. W. & Blurton-Jones, M. (2012). “Concise Review: Can Stem Cells Be Used to Treat or Model Alzheimer’s Disease?,” Stem Cells, 30, 2612—2618.
Publisher – Google Scholar
Cheng, K., Rai, P., Plagov, A., Lan, X., Kumar, D., Salhan, D., Rehman, S., Malhotra, A., Bhargava, K., Palestro, C. J., Gupta, S. & Singhal, P. C. (2013). “Transplantation of Bone Marrow-Derived MSCs Improves Cisplatinum-Induced Renal Injury through Paracrine Mechanisms,” Experimental and Molecular Pathology, 94 (3), 466-473.
Publisher – Google Scholar
Conde, C., Costa, V. & Tomaz. C. (2000). “Effects of Emotional Reactivity on Inhibitory Avoidance in the Elevated T-Maze,” Brazilian Journal of Medical and Biological Research, 33 (2), 233-236.
Publisher – Google Scholar
Ferreira, G. F. S. (2012). “Análise da Estrutura Fatorial do Comportamento Exploratório de Ratos em Modelos de Ansiedade, Depressão e Reconhecimento,” Unpublished Doctoral Dissertation. University of Brasília, Brasil. Pp106.
Publisher
Foudah, D., Redondo, J., Caldara, C., Carini, F., Tredici, G. & Miloso M. (2012). “Expression of Neural Markers by Undifferentiated Rat Mesenchymal Stem Cells,” Journal of Biomedicine & Biotechnology, 2012, Pp13.
Publisher – Google Scholar
Harsha, S. N. & Anilakumar, K. R. (2013). “Anxiolytic Property of Hydro-Alcohol Extract of Lactuca Sativa and Its Effect on Behavioral Activities of Mice,” Journal of Biomedical Research, 27 (1), 37-42.
Publisher – Google Scholar
Ibarretxe, G., Crende, O., Aurrekoetxea, M., Garcıa-Murga, V., Etxaniz, J. & Unda, F. (2012). “Neural Crest Stem Cells from Dental Tissues: A New Hope for Dental and Neural Regeneration,” Stem Cells International, 2012, Pp12.
Publisher – Google Scholar
Joyce, N., Annett, G., Wirthlin, L., Olson, S., Bauer, G. & Nolta, J. A. (2010). “Mesenchymal Stem Cells for the Treatment of Neurodegenerative Disease,” Regenerative Medicine, 5 (6), 933-946.
Publisher – Google Scholar
Katare, R., Stroemer, P., Hicks, C., Stevanato, L., Patel, S., Corteling, R., Miljan, E., Vishnubhatla, I., Sinden, J. & Madeddu, P. (2014). “Clinical-Grade Human Neural Stem Cells Promote Reparative Neovascularization in Mouse Models of Hindlimb Ischemia,” Arteriosclerosis Thrombosis and Vascular Biology, 34, 408-418.
Publisher – Google Scholar
Li, J., Ezzelarab, M. B. & Cooper, D. (2012). “Do Mesenchymal Stem Cells Function across Species Barriers? Relevance or Xenotransplantation,” Xenotransplantation, 19(5): 273—285.
Publisher – Google Scholar
Marchetti, V., Krohne, T. U., Friedlander, D. F. & Friedlander, M. (2010). “Stemming Vision Loss with Stem Cells,” The Journal of Clinical Investigation, 120 (9), 3012—3021.
Publisher – Google Scholar
Niedowicz, D. M., Nelson, P. T. & Murphy, P. M. (2011). “Alzheimer’s Disease: Pathological Mechanisms and Recent Insights,” Curr Neuropharmacol, 9, 674-684.
Publisher – Google Scholar
Okano, H., Sakaguchi, M., Ohki, K., Suzuki, N. & Sawamoto, K. (2007). “Regeneration of the Central Nervous System Using Endogenous Repair Mechanisms,” Journal of Neurochemistry, 102 (5), 1459—1465.
Publisher – Google Scholar
Oliveira, A., Hodges, H. & Rezaie, P. (2003). “Excitotoxic Lesioning of the Rat Basal Forebrain with S-AMPA: Consequent Mineralization and Associated Glial Response,” Experimental Neurology, V. 179, N.2, P. 127-138.
Publisher – Google Scholar
Page, K. J., Everitt, B. J., Robbins, T. W., Marston, H. M. & Wilkinson, L. S. (1991). “Dissociable Effects on Spatial Maze and Passive Avoidance Acquisition and Retention Following AMPA- and Ibotenic Acid-Induced Excitotoxic Lesions of the Basal Forebrain in Rats: Differential Dependence on Cholinergic Neuronal Loss,” Neuroscience, 43(2-3), 457-472.
Publisher – Google Scholar
Prince, M., Bryce, R. & Ferri, C. (2011). “World Alzheimer Report 2011: The Benefits of Early Diagnosis and Intervention,” http://www.alz.co.uk. Accessed 25 February 2014.
Publisher
Roux, S., Leotot, J., Chevallier, N., Bierling, P. & Rouard, H. (2011). “Mesenchymal Stromal Cells: Biological Properties and Clinical Prospects,” Transfusion Clinique et Biologique, 18 (1), 1-12.
Publisher – Google Scholar
Smith, E. J., Stroemer, P., Gorenkova, N., Nakajima, M., Crum, W. R., Tang, E., Stevanato, L., Sinden, J. D. & Modo, M. (2012). “Implantation Site and Lesion Topology Determine Efficacy of a Human Neural Stem Cell Line in a Rat Model of Chronic Stroke,” Stem Cells, 30, 785-796.
Publisher – Google Scholar
Weintraub, S., Salmon, D., Mercaldo, N., Ferris, S., Graff-Radford, N. R., Chui, H., Cummings, J., Decarli, C., Foster, N., Galasko, D., Peskind, E., Dietrich, W., Beekly, D., Kukull, W. A. & Morris, J. C. (2009). “The Alzheimer’s Disease Center’s Uniform Data Set (UDS): The Neuropsychological Test Battery,” Alzheimer disease and Associated Disorders,23 (2), 91-101.
Publisher – Google Scholar
Wider, C. & Wszolek, Z. K. (2008). “Etiology and Pathophysiology of Frontotemporal Dementia, Parkinson Disease and Alzheimer Disease: Lessons from Genetic Studies,” Neurodegenerative Diseases, 5,122-125.
Publisher – Google Scholar
Zangrossi, H. & Graeff, F. G. (1997). “Behavioral Validation of the Elevated T-Maze: A New Animal Model of Anxiety,”Brain Research Bulletin, 44 (1), 1-5.
Publisher – Google Scholar