Introduction
There is a wide range of bone grafting techniques used in the practice of implant dentistry. Allografts and xenografts have an advantage in that they do not require a donor site like autogenous bone grafts (Morton et al 2013). However concerns remain about their absolute non-infectivity. Furthermore those with particular ethnic and religious beliefs are unsuitable for bone grafting with these materials (Wenz 2001, Hämmerle et al 2012).
On-going research in the field of bone grafting strives for a safe, predictable, efficient material and technique that minimises morbidity for the patient while optimising the potential benefits (Buser et al 2013). While traditional autogenous bone grafting techniques do offer considerable biological advantages for patients, they are also associated with increased morbidity and likelihood of complications (Misch and Misch-Dietsh 2008). Alloplastic bone grafting materials do address many problems associated with the other non-autogenous materials and therefore have a distinct advantage (von Arx and Buser 2006, Miron et al 2013). However, like other autogenous alternatives concerns have been raised about the amount and the rate at which they are replaced by the recipient’s natural bone (Donos et al 2008, Jensen et al 2013).
Brushite bone cement has been shown to behave favourably in this respect and chondroitin sulphate has been shown to further enhance this process in other bone grafting materials (Zou et al 2004, Scabbia and Trombelli 2004, Theiss et al 2005, Tamimi et al 2005, Schneiders et al 2008, Schneiders et al 2009, Tamimi et al 2012, Engstrand et al 2013).
Aim
To determine whether cells can grow on brushite cement and on brushite cement containing chondroitin-6-suphate
Objectives
To assess the cellular response of a continuous cell line to brushite cement containing varying concentrations of chondroitin-6-sulphate
- The MG-63 osteosarcoma cell line will be utilised
- Cell attachment will be investigated using scanning electron microscopy (SEM)
- Cellular proliferation will be analysed using the AlamarBlue® assay
Settings and Design
All the preparatory and investigatory work was undertaken in the cell culture laboratory (Biomaterials and Tissue Engineering Division at the Eastman Dental Institute, University College London, UK) using strict aseptic techniques. Equipment and surfaces were disinfected prior to use with Klercide CR® and 70 per cent ethanol. New consumable pipettes, test tubes, bottles, flasks and instrumentation were used where possible and sterilisation was performed using a 123ºC for 120 minutes cycle in a 200/25LE Autoclave (Boxer Laboratory Equipment Ltd., Ware, UK). All cell work was undertaken in a “Class 2” biological safety cabinet (NU-425-400E Series 21: NuAire, Plymouth, USA). This biological safety cabinet was operational at a pressure of 15-20mm H2O, with an inflow of 0.46ms-1 creating a down-flow of 0.38ms-1. A Steristrom 2537å (Coast-Air, London, UK) was used for ultraviolet light sterilisation. Cells were incubated at 37ºC in a five per cent CO2 DH Autoflow Air-Jacketed Incubator (NuAire, Plymouth, USA) and culture photography was performed using an Olympus CK-2 digital camera at x4 and x10 magnification (Olympus, Southall, UK).
Materials
Monocalcium phosphate monohydrate (MCPM) powder with median particle size of 62µm (Rhodia, Birmingham, UK)
Beta-tricalcium phosphate (β-TCP) powder with median particle size of 11µm (Rhodia, Birmingham, UK)
Chondroitin-6-sulphate powder derived from shark cartilage (Sigma-Aldrich, Gillingham, UK)
Citric acid crystals (Fisher, Loughsborough, UK)
Distilled water
Dulbecco’s Modified Eagle’s Medium – low glucose (DMEM)
Penicillin-streptomycin (100x) (100U penicillin and 10µg streptomycin ml-1)
Foetal bovine serum (FBS)
Phosphate buffered saline (PBS)
Trypsin-EDTA (1x) (0.05/0.02 % in Dulbecco’s-PBS)
Trypan Blue® 0.4 % solution (Sigma-Aldrich, Gillingham, UK)
MG-63 osteosarcoma cells
Thermanox® coverslips (13mm diameter) (Agar Scientific, Stansted, UK)
Alamar Blue® proliferative assay (AbD Serotec, Oxford, UK)
Klercide CR® (Sigma-Aldrich, Gillingham, UK)
Ethanol
Glutaraldehyde (3% in 0.1M cacodylate buffer) (Agar Scientific, Stansted, UK)
Hexamethyldisilazane (HMDS) (TAAB Laboratories, Reading, UK)
Conducting carbon cement (Neubauer Chemikalien, Munster, Germany)
Polaron E5000 gold/palladium sputter coating (Quorum Technologies, Newhaven, UK)
Methods
Production of Brushite Disks Containing Condroitin-6-Sulphate
The constituent materials for the production of the cement disks were weighed cumulatively using a four-figure electronic laboratory balance as see in Figure 1. The formulations, as seen in Table 1, were calculated to give zero, five and ten per cent chondroitin-6-sulphate relative to the total mass of the powder materials included, namely the chondroitin-6-sulphate, MCPM and β-TCP.
Citric acid was used as a retardant in the setting reaction that allows good handling properties of the setting cement. 400mM citric acid was prepared by adding 7.84g citric acid crystals to 100ml distilled H2O.
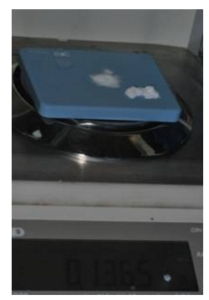
Figure 1: Materials on the Electronic Balance
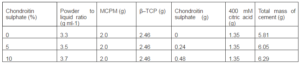
Table 1: Formulations for the Production of the Cement Disks
After weighing, a spatula was used to mix the powders first to ensure homogeneity and then the 400mM citric acid was added to the mix. This paste was mixed for one minute. The mixture was placed in 11mm diameter metal rings (2mm height) on an acetate sheet with a glass slab below. Another acetate sheet with a second glass slab was placed on top and pressure was applied until the upper of the metal ring was apparent (Figure 2).
The following reaction resulted in the cement disks incorporating the desired percentages of chondroitin-6-sulphate:
β-Ca3(PO4)2 + Ca(H2PO4)2-H20 + 7H2O à 4CaHPO4-2H20
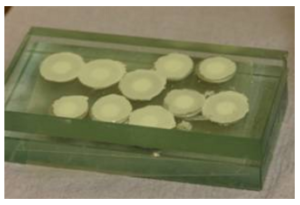
Figure 2: Example of 11mm Diameter Cement Disks Setting
For each concentration of chondroitin-6-sulphate a minimum of two mixtures were prepared and the cement disks were stored in hermetically sealed polyethylene bags after retrieval. These were stored at 4ºC, as is the chondroitin-6-sulphate manufacturer’s recommendation.
Before these cement disks were used in the experimental stages they were first sterilized using ultraviolet light in the Steristrom 2537å. The disks were first sprayed lightly with 70 per cent ethanol, allowed to air dry in the biological safety cabinet for five minutes and placed 8cm from the light source for 60 minutes. Following this the disks were aseptically turned and this procedure was repeated.
Following the sterilization two cement disks from each of the three goup were placed in three separate 20ml tubes of tissue culture medium and stored at 4ºC. In order to assess the surfaces of the cement disks after immersion in tissue culture medium one disk from each tube was removed after 24 hours and the others after ten days for imaging by the SEM.
Preparation of Tissue Culture Medium
Before experimental work fresh tissue culture medium was prepared by adding 50ml FBS and 10ml penicillin-streptomycin to 440ml DMEM in the biological safety cabinet. Both the FBS and penicillin-streptomycin were thawed from frozen to 37ºC in a water bath prior to mixing. This medium was heated to 37ºC before cell work and stored at 4ºC.
Preparation of MG-63 Cells
Human MG-63 osteosarcoma cells were resurrected from storage in liquid nitrogen at less than -130ºC and at an initial known approximate concentration of 1,000,000 cells ml-1 (Figure 3). The vial was brought to 37ºC and transferred to a cell culture flask with a 75cm2 surface area for cell attachment. Medium was added initially one drop at a time at an approximate rate of 1ml in the first minute (Figure 4). In total 20ml of medium was added to the flask and cells were visualised under the microscope. The flask was placed in the incubator at 37ºC and checked on a daily basis to see if the cells were confluent. The medium was changed as required.
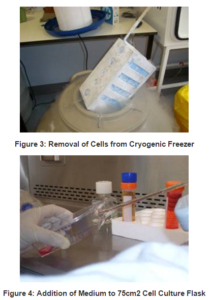
The cells were then washed with PBS (10ml twice) and split using 5ml trypsin-EDTA when necessary. Cells were verified to be split under the microscope and 10ml medium was added into the trypsinized live cells to inhibit the trypsinization, followed by centrifuging at 200g for five minutes. The supernatant was decanted without disturbing the cell pellet that had formed. A further 10ml medium was added and the tube vigorously vibrated to disperse the cells in the medium. Counting of the cells was accomplished using Trypan Blue® and a haemocytometer (Neubauer Chemikalien, Munster, Germany). Haemocytometer slide with monolayer of cells were placed under the microscope and the viable unstained cells were counted in the 1mm2 grid (n) at 10x objective magnification. This 1mm2 grid area of the haemocytometer chamber is known to have a volume of 0.1mm3 (v), which allowed the cell concentration (c) to be calculated using the formula below:
c = n/v
Each cell count was done in duplicate and the values averaged for c. Differing pre-determined amounts of fresh medium were added to the cell solution of calculated concentration. This gave pre-determined different concentrations of cells in new tubes as were required for the cell work.
Seeding of MG-63 Cells and Schedule of Experimental Work
For the investigatory work a 24-well plate was set-up with quadruplicate wells for:
Plain tissue culture medium (triplicate wells only)
Thermanox® coverslips
0% chondroitin-6-sulphate brushite cement disks
5% chondroitin-6-sulphate brushite cement disks
10% chondroitin-6-sulphate brushite cement disks
In 16 of the 19 wells cells were seeded by the addition of medium with a cell concentration of 10,000 cells 50µl-1. A 50µl meniscus was pipetted onto the centre of each coverslip and cement disk, and then the plate was placed in the incubator for two hours. At this time medium was placed in each well in order to have a total of 1ml solution in each well. In the remaining three wells 1ml of plain tissue culture medium was added to establish control wells for the background Alamar Blue® fluorescence readings. The plate was placed in the incubator at 37ºC. After 24 hours, at the time of the first proliferation readings, the fourth coverslip and cement disk from each of the four groups was removed for SEM analysis. This left triplicate wells for each group and Alamar Blue® colorimetric and fluorescence proliferation testing was undertaken at days one, three and six, as seen in Figure 5. Following the last testing a further coverslip and cement disk from each of the four groups was removed for endpoint SEM analysis.
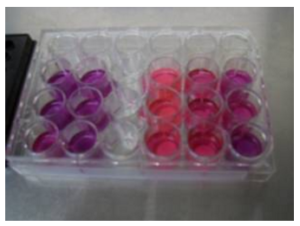
Figure 5: Triplicate Wells for Each Group in 24-Well Plate
Alamar Blue® Proliferative Assay
Alamar Blue® is a redox indicator and used quantitatively to measure cellular proliferation (Nakayama et al., 1997 and Chen et al., 2008). A standard curve was created by seeding increasing concentrations of cells in triplicate wells in a 24-well plate as per Table 2. All the cells for each well were seeded initially as a 50µl meniscus to establish an even seeding surface area in each well and placed in the incubator for two hours at 37ºC. Medium was then added so each well contained 1ml of cell solution and this was placed in the incubator for a further 22 hours.
At this stage 100µl Alamar Blue® was added to each well and this plate was placed back in the incubator for two hours. Duplicate 100µl samples were removed from each well using 200µl pipettes and transferred into separate wells in a black 96-well plate for fluorescence readings. New 200µl pipettes tips were used for sample extraction from each well. The duplicate sample readings of each well were averaged, as were the readings of the triplicate wells for each initial seeding density. The averaged background Alamar Blue® fluorescence reading was also calculated in the same way and this was taken from the results for each initial seeding density. These data were used to plot a standard curve of fluorescence readings against cell counts for MG-63 cells.
Table 2: Standard Curve Cell Counts
For the proliferation testing of the MG-63 cells on the Thermanox® coverslips as the control and the brushite cement disks with differing concentrations of chondroitin-6-sulphate, 100µl Alamar Blue® was added to each well at the desired time points. These were days one, three and six. Following a subsequent incubation period of two hours duplicate 100µl samples were removed from each well with a 200µl pipette and placed in separate wells in a black 96-well plate for fluorescence readings. The 200µl pipettes tips were discarded after use in each well and replaced with a new one.
Following the sampling of each well for fluorescence readings the medium was removed and subsequently replaced with 1ml of new medium per well. Before this new medium was added, each well was twice flushed with and emptied of new medium in order to remove traces of Alamar Blue®. The removal of medium from the wells was accomplished using new glass pipette tips for each well that were connected to a motorised aspirator device.
Fluoroscopy readings were taken and analysed using a Fluoroskan Ascent Fluorimeter (Figure 6) and the accompanying Ascent Software Version 2.4 (Lab Systems, Altrincham, UK). The excitation filter was set at 530nm, the emission filter at 590nm and the integration time at 20ms with a normal beam size.
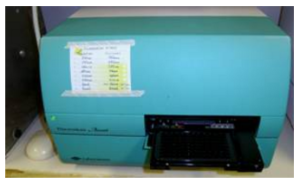
Figure 6: Fluorimeter with Black 96-Well Plate
The relative cell viability was calculated at each time. The Thermanox® coverslip controls were used as the standard. For each reading of fluorescence the background acellular fluorescence was first subtracted and the percentage relative cell viability calculated as per the following formula:
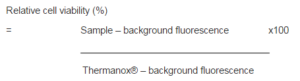
Scanning Electron Microscopy
Immediately before fixation the Thermanox® coverslips and cement disk specimens were rinsed in fresh culture medium. All specimens were fixed for 24 hours in glutaraldehyde (3% in 0.1M cacodylate buffer) before dehydration at room temperature in a series of graded ethanol concentrations. These concentrations and accompanying drying times are shown in Table 3. At this stage these critical point dry specimens were then immersed in HMDS for three minutes before being removed and allowed to air dry in a biological safety cabinet for one hour. The specimens were then mounted onto conducting carbon cement before being sputter coated with gold/palladium. Imaging was undertaken with the JEOL 5410LV SEM (JEOL UK Ltd, Welwyn Garden City, UK) operating at 15kV. The images were captured at a magnification range of x35 and x3,500.
Table 3: SEM Drying Protocol
Data Collection and Analysis
All numerical data were recorded using Microsoft Excel®. Subsequent mathematical analysis was undertaken using this software. Images recorded from the SEM investigations were converted into the jpeg format for storage and presentation.
The in vitro proliferation tests were performed on triplicate samples of each material. The Alamar Blue® sample from each well was taken in duplicate for the main experimental work and each set of these two figures were averaged before analysis. Each set of triplicate results per material per time point were averaged. The “background” Alamar Blue® was also calculated using the above method. This was subtracted from the results of the Thermanox® coverslips as the control and the brushite cement disks with differing concentrations of chondroitin-6-sulphate. The standard deviation for each triplicate result was determined and presented with the results as an error bar.
Results
Alamar Blue® Proliferation Assay
The Alamar Blue® fluorescence readings for the differing cell concentrations after 24 hours are presented in Table 4. These have been corrected for the background Alamar Blue® fluorescence readings as measured from acellular wells in the same conditions. The triplicate well results have been averaged and the standard deviation for each is shown as an error bar in Figure 7.
Table 4: Standard Curve Alamar Blue® Fluorescence Readings
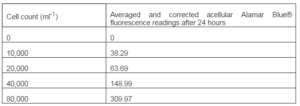
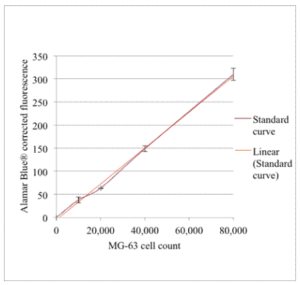
Figure 7: MG-63 Alamar Blue® Fluorescence Standard Curve (N=3 for Each Data Point and Error Bars Present ± Standard Deviation)
The straight line fit for this curve gave R² = 0.9977. The relationship between MG-63 cell numbers and corrected Alamar Blue® fluorescence readings can be calculated from the straight-line formula:
y = 0.0039x – 4.7048
The MG-63 cellular proliferative results of the cements are displayed in Figure 8. They are relative to the cellular proliferative results observed on the Thermanox® coverslips, which acted as the control. For each time point the corrected and averaged results for the Thermanox® are displayed as 100 per cent and the other corrected and averaged results are relative to this. The standard deviation for each triplicate result is shown as the error bar.
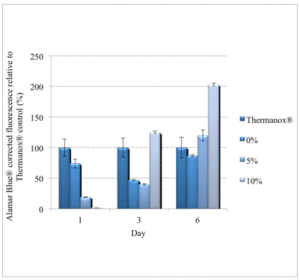
Figure 8: Main Experimental Proliferative Results for the Three Groups of Brushite Cements with Chondroitin-6-Sulphate Relative to Thermanox® as the Control (N=3 for Each Result and Error Bars Present ± Standard Deviation)
Scanning Electron Microscopy
The qualitative SEM studies of the plain brushite cement disks (0%) and those containing chondroitin-6-sulphate (5% and 10%) are shown in Figures 9 to 12 at different time points. “Day 0” refers to dry and unseeded disks that underwent SEM analysis without ever being immersed in tissue culture medium. “Day 1” and “Day 6” refer to disks that were immersed in tissue culture medium in sterile conditions at 37ºC without being seeded with MG-63 cells. The magnification for each image is displayed in each figure. Degradation of the disks seemed to increase with immersion time.
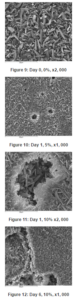
Cellular Attachments from Experimental Work
There was not any evidence of bacteria from either time point of SEM imaging, “Day 1” or “Day 6”. The SEM “Control” Thermanox® coverslip images is displayed in Figures 13 showing cellular proliferation after 24 hours. Figures 14 to 17 sequentially display the SEM images of the brushite cements with different concentrations of chondroitin-6-sulphate at the two time points.
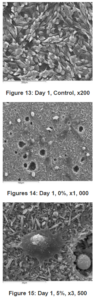
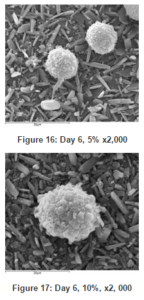
Discussion
The general way in which the experimental work was conducted was as per protocol in the cellular laboratory using tried and tested techniques as described by Freshney (2005). The production of the cements was carried out in the same fashion as done previously by Smith (2008) for work to characterise these materials. The continuous MG-63 osteosarcoma cell line was used as this has been claimed and proven to be a relevant experimental model for investigating osteoblast function. Clover and Gowen (1994) reported that this cell line in vitro produces appropriate osteoblastic phenotype representation of both cellular adhesion and proliferation.
The Alamar Blue® proliferative assay used is a reliable method of assessing living cell metabolism and obtaining an indirect yet real time quantification of cellular proliferation (Nakayama et al 1997). Moreover it is a non-destructive, sensitive and non-radioactive homogenous assay. Alamar Blue® is a commonly used assay and has been previously used to assess proliferation of MG-63 cells on other alloplastic bone graft materials in vitro (Chen et al 2008).
Using the meniscus technique, where the initial 10,000 cells per well were seeded on top of the Thermanox® or cement disks as a 50µm meniscus, allowed for cell attachment in the initial period of two hours. Cells could only attach to approximately a 10mm diameter surface area of the material being studied and not to the surrounding well (Figures 18 and 19).
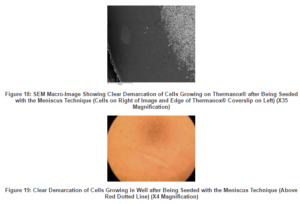
Both the Alamar Blue® proliferative results relative to the Thermanox® control at day three and six, and the SEM imaging at day six indicate that, there was a time related increase in cellular activity on the cements with chondroitin-6-sulphate.
At day six the Thermanox® coverslip displayed areas of mutli-layered cellular confluence and cell spreading. There was comparable cellular growth on the brushite cement disk containing chondroitin-6-sulphate, Dark areas indicating well attached, thin spreading cells as well as brighter clusters indicating cells that are yet to spread, were displayed and this activity was present across the entire surface of many disk (Figures 15 to 17).
There was a two-fold increase in cellular activity relative to the Thermanox® control at day six for the ten per cent samples (Figure 8). The SEM imaging shows that while there are abundant MG-63 osteosarcoma cells present, they were rounded (Figure 17). This is in contrast to flattened cells with evidence of cellular spread across the cements, as can be seen in Figure 15. While there seems to be a time related increased affinity for the cements as the concentration of chondroitin-6-sulphate increases, the reason for this lack of frank cellular attaching and spreading is unclear. Similar observations were reported by Jamshidi et al (2013). It may be due to the acidic nature of, or another factor related to, the chondroitin-6-sulphate constituent (Young et al 2008, Alkhraisat et al 2010). Furthermore there may be a link to the surface degradation that was evident in the acellular studies (Figure 12). Possible leaching of chondroitin-6-sulphate could result in a change to the mechanical properties of the brushite cement as a whole (Alkhraisat et al 2008, Zhao et al 2010).
This was the first time that cellular growth studies have been undertaken on these particular materials. It did become apparent during the evaluation of the results that the acidic nature of the materials could be an influence in the observations. In repeats of these experiments it would be very useful to evaluate pH readings at various stages. These data would have been very useful and could have possibly partially helped in the interpretation of some of the results. Measuring the pH at different time points could be done in a non-invasive fashion and would definitely be recommended in future experiments. Furthermore, while the use of MG-63 osteosarcoma cell are routinely used to investigate osteoblast adhesion and proliferation, it is known that there are differences such as increased enzyme activity may occur relative to when human bone derived calls are used.
In all surgical procedures one of the essential requirements is the predictability of the successful outcome of the procedure. Alveolar bone grafting procedures aim to facilitate or promote bone healing, with the restoration of morphology and function (Chiapasco et al 2006, Albrektsson et al 2013). The bone generated should have adequate dimensions to place prosthodontically driven implants (Donos et al 2008).
Research into improvements of the clinical effectiveness of synthetic bone grafting materials, such as calcium phosphate cements, is definitely warranted as these materials offer many advantages over other grafting materials that are commonly used (Hämmerle and Jung 2003). While autogenous bone grafts have long been deemed as the “gold standard” for bone augmentation (Buser et al 1998, Palmer 1999, del Fabbro et al 2004, Aghaloo and Moy 2007, Esposito et al 2008), they are associated with some drawbacks such as donor site morbidity (von Arx and Buser 2006). While membranes do enhance the quantity and quality of the generated tissues (Lang et al 1994, Nyman and Lang 1994, Hämmerle and Lang 2001, Zitzmann et al 2001, Hämmerle et al 2002, Hämmerle and Jung 2003), developments that result in quicker resorption of the graft material with subsequent natural bone deposition are strived for in the field of implant dentistry. These materials must be at least osteoconductive and undergo complete remodelling in a predictable time frame (Buser et al 1998, Zitzmann et al 2001, Bohner et al 2005, Chiapasco et al 2006, Aghaloo and Moy 2007, Young et al 2008). For the placement of dental implants the ideal scenario is for the graft material to be replaced by natural bone that should function in a clinically predictable way, and not by bone-like substances of arguably poorer quality.
Conclusions
This research has conclusively shown that bone cells can grow on brushite cement alone and on brushite cements containing varying concentrations of chondroitin-6-sulphate.
While, after six days in vitro, the MG-63 osteosarcoma cells did preferentially attach to the chondroitin-6-sulphate containing brushite cements relative to the Thermanox® control or the brushite without chondroitin-6-sulphate, it must be determined why the cells failed to actively spread over the surfaces of these cements, instead remaining rounded and coherent.
These results indicate that additional research is warranted on the chemistry of these cements when in contact with body fluids. It must also be established why rapid cell proliferation was initially delayed on the 10% chondroitin-6-sulphate brushite cement disks, relative to with 0% and 5% chondroitin-6-sulphate. Particular focus should be given to the effect of pH changes that may occur, as well as functional cellular testing and investigating the mechanical properties of the set cements.
References
Aghaloo, T. L. & Moy, P. K. (2007). “Which Hard Tissue Augmentation Techniques are the Most Successful in Furnishing Bony Support for Implant Placement?,” International Journal of Oral and Maxillofacial Implants, 22 (Suppl): 49-70.
Publisher – Google Scholar
Albrektsson, T., Buser, D. & Sennerby, L. (2012). “Crestal Bone Loss and Oral Implants,” Clinical Implant Dentistry and Related Research, Dec; 14(6):783-91.
Publisher – Google Scholar
Alkhraisat, M. H., Marino, F. T., Retama, J. R., Jerez, L. B. & López-Cabarcos, E. (2008). “Beta-Tricalcium Phosphate Release from Brushite Cement Surface,” Journal of Biomedical Materials Research Part A, 84 (3): 710-7.
Publisher – Google Scholar
Alkhraisat, M. H., Rueda, C., Jerez, L. B., Tamimi Mari-o, F., Torres, J., Gbureck, U. & Lopez Cabarcos, E. (2010). “Effect of Silica Gel on the Cohesion, Properties and Biological Performance of Brushite Cement,” Acta Biomaterialia.Jan; 6(1):257-65.
Publisher – Google Scholar
Bohner, M., Gbureck, U. & Barralet, J. E. (2005). “Technological Issues for the Development of More Efficient Calcium Phosphate Bone Cements: A Critical Assessment,” Biomaterials, 26 (33): 6423-9.
Publisher – Google Scholar
Buser, D., Chappuis, V., Bornstein, M. M., Wittneben, J. G., Frei, M. & Belser, U. C. (2013). Long-Term Stability of Contour Augmentation with Early Implant Placement Following Single Tooth Extraction in the Esthetic Zone a Prospective, Cross-Sectional Study in 41 Patients with a 5- to 9-Year Follow-up. Journal of Periodontology Jan 24. [Epub Ahead of Print]
Publisher – Google Scholar
Buser, D., Hoffmann, B., Bernard, J. P., Lussi, A., Mettler, D. & Schenk, R. K. (1998). “Evaluation of Filling Materials in Membrane–Protected Bone Defects. A Comparative Histomorphometric Study in the Mandible of Miniature Pigs,”Clinical Oral Implants Research, 9 (3): 137-50.
Publisher – Google Scholar
Chen, Q. Z., Efthymiou, A., Salih, V. & Boccaccini, A. R. (2008). “Bioglass-Derived Glass-Ceramic Scaffolds: Study of Cell Proliferation and Scaffold Degradation in Vitro,” Journal of Biomedical Materials Research Part A, 84 (4): 1049-60.
Publisher – Google Scholar
Chiapasco, M., Zaniboni, M. & Boisco, M. (2006). “Augmentation Procedures for the Rehabilitation of Deficient Edentulous Ridges with Oral Implants,” Clinical Oral Implants Research, 17 (Suppl 2): 136-59.
Publisher – Google Scholar
Clover, J. & Gowen, M. (1994). “Are MG-63 and HOS TE85 Human Osteosarcoma Cell Lines Representative Models of the Osteoblastic Phenotype?,” Bone, 15 (6): 585-91.
Publisher – Google Scholar
Del Fabbro, M., Testori, T., Francetti, L. & Weinstein, R. (2004). “Systematic Review of Survival Rates for Implants Placed in the Grafted Maxillary Sinus,” International Journal of Periodontics and Restorative Dentistry, 24 (6): 565-77.
Publisher – Google Scholar
Donos, N., Mardas, N. & Chadha, V. (2008). “Clinical Outcomes of Implants Following Lateral Bone Augmentation: Systematic Assessment of Available Options (Barrier Membranes, Bone Grafts, Split Osteotomy),” Journal of Clinical Periodontology, 35 (Suppl 8): 173-202.
Publisher – Google Scholar
Engstrand, J., Persson, C. & Engqvist, H. (2013). “The Effect of Composition on Mechanical Properties of Brushite Cements,” Journal of the Mechanical Behavior of Biomedical Materials. Sep 5;29C:81-90.
Publisher – Google Scholar
Esposito, M., Grusovin, M. G., Kwan, S., Worthington, H. V. & Coulthard, P. (2008). “Interventions for Replacing Missing Teeth: Bone Augmentation Techniques for Dental Implant Treatment,” Cochrane Database of Systematic Reviews, 16 (3): CD003607.
Publisher – Google Scholar
Freshney, R. I. (2005). Culture of Animal Cells: A Manual of Basic Techniques, New Jersey: Wiley.
Publisher – Google Scholar
Hämmerle, C. H. & Lang, N. P. (2001). “Single Stage Surgery Combining Transmucosal Implant Placement with Guided Bone Regeneration and Bioresorbable Materials,” Clinical Oral Implants Research, 12 (1): 9-18.
Publisher – Google Scholar
Hämmerle, C. H. F., Araújo, M. G. & Simion, M. (2012). Osteology Consensus Group 2011. “Evidence-Based Knowledge on the Biology and Treatment of Extraction Sockets,” Clinical Oral Implants Research. Feb;23 Suppl 5:80-2.
Publisher – Google Scholar
Hämmerle, C. H. F., Jung, R. E. & Feloutzis, A. (2002). “A Systematic Review of the Survival of Implants in Bone Sites Augmented with Barrier Membranes (Guided Bone Regeneration) in Partially Edentulous Patients,” Journal of Clinical Periodontology, 29 (Suppl 3): 226-31.
Publisher – Google Scholar
Hämmerle, C. H. F. & Jung, R. E. (2003). “Bone Augmentation by Means of Barrier Membranes,” Periodontology 2000,33: 36-53.
Publisher – Google Scholar
Jamshidi, P., Bridson, R. H., Wright, A. J. & Grover, L. M. (2013). “Brushite Cement Additives Inhibit Attachment to Cell Culture Beads,” Biotechnology and Bioengineering. May;110(5):1487-94.
Publisher – Google Scholar
Jensen, S. S., Aaboe, M., Janner, S. F. M., Saulacic, N., Bornstein, M. M., Bosshardt, D. D. & Buser, D. (2013). “Influence of Particle Size of Deproteinized Bovine Bone Mineral on New Bone Formation and Implant Stability after Simultaneous Sinus Floor Elevation: A Histomorphometric Study in Minipigs,” Clinical Implant Dentistry and Related Research. Jun 23.
Publisher – Google Scholar
Lang, N. P., Hämmerle, C. H. F., Brägger, U., Lehmann, B. & Nyman, S. R. (1994). “Guided Tissue Regeneration in Jawbone Defects Prior to Implant Placement,” Clinical Oral Implants Research, 5 (2): 92-7.
Publisher – Google Scholar
Miron, R. J., Gruber, R., Hedbom, E., Saulacic, N., Zhang, Y., Sculean, A., Bosshardt, D. D. & Buser, D. (2013). “Impact of Bone Harvesting Techniques on Cell Viability and the Release of Growth Factors of Autografts,” Clinical Implant Dentistry and Related Research. Aug; 15(4):481-9.
Publisher – Google Scholar
Misch, C. E. & Misch-Dietsh, F. (2008). ‘Key to Bone Grafting and Bone Grafting Materials,’ In: CE Misch, Ed.,Contemporary Implant Dentistry. St. Louis: Mosby Elsevier.
Morton, D., Chen, S. T., Martin, W. C., Levine, R. & Buser, D. (2013). Consensus Statements and Recommended Clinical Procedures Regarding Optimizing Esthetic Outcomes in Implant Dentistry, The International Journal of Oral & Maxillofacial Implants. Aug 15.
Publisher – Google Scholar
Nakayama, G. R., Caton, M. C., Nova, M. P. & Parandoosh, Z. (1997). “Assessment of the Alamar Blue Assay for Cellular Growth and Viability in Vitro,” The Journal of Immunological Methods, 204 (2): 205-8.
Publisher – Google Scholar
Nyman, S. R. & Lang, N. P. (1994). “Guided Tissue Regeneration and Dental Implants,” Periodontology 2000, 4: 109-18.
Publisher – Google Scholar
Palmer, P. & Palmer, R. (1999). “Dental Implants 8. Implant Surgery to Overcome Anatomical Difficulties,” British Dental Journal, 187 (10): 532-40.
Publisher – Google Scholar
Scabbia, A. & Trombelli, L. (2004). “A Comparative Study on the Use of a HA/Collagen/Chondroitin Sulphate Biomaterial (Biostite) and a Bovine-Derived HA Xenograft (Bio-Oss) in the Treatment of Deep Intra-Osseous Defects,” Journal of Clinical Periodontology, 31 (5): 348-55.
Publisher – Google Scholar
Schneiders, W., Reinstorf, A., Biewener, A., Serra, A., Grass, R., Kinscher, M., Heineck, J., Rehberg, S., Zwipp, H. & Rammelt, S. (2009). “In Vivo Effects of Modification of Hydroxyapatite/Collagen Composites with and without Chondroitin Sulphate on Bone Remodeling in the Sheep Tibia,” Journal of Orthopaedic Research, 27 (1): 15-21.
Publisher – Google Scholar
Schneiders, W., Reinstorf, A., Ruhnow, M., Rehberg, S., Heineck, J., Hinterseher, I., Biewener, A., Zwipp, H. & Rammelt, S. (2008). “Effect of Chondroitin Sulphate on Material Properties and Bone Remodelling around Hydroxyapatite/Collagen Composites,” Journal of Biomedical Materials Research Part A, 85 (3): 638-45.
Publisher – Google Scholar
Smith, S. F. (2008). ‘Production and Characterisation of Brushite Bone Cements Containing Chondroitin-6-Sulphate,’ M.Sc. Thesis, Eastman Dental Institute, University College London.
Tamimi, F., Kumarasami, B., Doillon, C., Gbureck, U., Le Nihouannen, D., Cabarcos, E. L. & Barralet, J. E. (2008). “Brushite-Collagen Composites for Bone Regeneration,” Acta Biomaterialia, 4 (5): 1315-21.
Publisher – Google Scholar
Tamimi, F., Sheikh, Z. & Barralet, J. (2012). “Dicalcium Phosphate Cements: Brushite and Monetite,” Acta Biomaterialia,Feb;8 (2):474-87.
Publisher – Google Scholar
Theiss, F., Apelt, D., Brand, B., Kutter, A., Zlinszky, K., Bohner, M., Matter, S., Frei, C., Auer, J. A. & Von Rechenberg, B. (2005). “Biocompatibility and Resorption of a Brushite Calcium Phosphate Cement,” Biomaterials, 26 (21): 4383-94.
Publisher – Google Scholar
Von Arx, T. & Buser, D. (2006). “Horizontal Ridge Augmentation Using Autogenous Block Grafts and the Guided Bone Regeneration Technique with Collagen Membranes: A Clinical Study with 42 Patients,” Clinical Oral Implants Research,17 (4): 359-66.
Publisher – Google Scholar
Wenz, B., Oesch, B. & Horst, M. (2001). “Analysis of the Risk of Transmitting Bovine Spongiform Encephalopathy through Bone Grafts Derived from Bovine Bone,” Biomaterials, 22 (12): 1599-606.
Publisher – Google Scholar
Young, A. M., Ng, P. Y. J., Gbureck, U., Nazhat, S. N., Barralet, J. E. & Hofmann, M. P. (2008). “Characterization of Chlorhexidine-Releasing, Fast-Setting, Brushite Bone Cements,” Acta Biomaterialia, 4 (4): 1081-8.
Publisher – Google Scholar
Zhao, X., Olsen, I., Li, H., Gellynck, K., Buxton, P. G., Knowles, J. C., Salih, V. & Young, A. M. (2010). “Reactive Calcium-Phosphate-Containing Poly (Ester-Co-Ether) Methacrylate Bone Adhesives: Chemical, Mechanical and Biological Considerations,”Acta Biomaterialia, Mar; 6(3):845-55.
Publisher – Google Scholar
Zitzmann, N. U., Schärer, P. & Marinello, C. P. (2001). “Long-Term Results of Implants Treated with Guided Bone Regeneration: A 5-Year Prospective Study,” International Journal of Oral and Maxillofacial Implants, 16 (3): 355-65.
Publisher – Google Scholar
Zou, X. H., Foong, W. C., Cao, T., Bay, B. H., Ouyang, H. W. & Yip, G. W. (2004). “Chondroitin Sulfate in Palatal Wound Healing,” Journal of Dental Research, 83 (11): 880-5.
Publisher – Google Scholar