Introduction
There is increasing interest in the study of bacteriophages of Erwinia amylovora (Gammaproteobacteria; Enterobacteriales), the causative agent of fire blight of Rosaceae plants, one of the most devastating plants diseases, that is of particular economic importance in the cultivation of apple, pear, and quince. Phage application has been shown to reduce infection of fire blight on apple flowers of potted apple trees by 56% and thereby compared well with the antibiotic streptomycin (Boulé et al., 2011). Our previous experiments have shown that phages were able to keep the population size of E. amylovora bacteria on quince seedlings artificially infected with fire blight at an asymptomatic level (Samoilova and Terteak, 2012).
- amylovora bacteria are infected by tailed prokaryote viruses falling into the three basic families Myoviridae, Siphoviridae, and Podoviridae (Schnabel and Jones, 2001; Gill et al., 2003; Boulé et al., 2011; Born et al., 2011; Roach et al., 2013) comprising 24.8%, 57.3%, and 14.2 % of all E. amylovora viruses, respectively (Ackermann and Prangishvili, 2012). The host range of these phage families depends on exopolysaccharide (EPS) capsules of E. amylovora cells (Müller et al., 2011b). Myoviridae phages are adapted to hosts that produce lower levels of EPS and host-produced levan, whereas Podoviridae phages are adapted to hosts that produce high levels of EPS and are dependent on host-produced amylovoran for pathogenesis (Roach et al., 2013). It has, for instance, been demonstrated that L1-like small podoviruses isolated on E. amylovora strain CFBP 1430 were unable to infect E. amylovora strain 4/82 which produces smaller amounts of EPS (Born et al., 2011).
Amylovoran contributes to bacterial movement in planta (Bogs and Geider, 2000) and together with levan is required for biofilm formation by E. amylovora. The absence of amylovoran production is accompanied by a reduction in solid surface attachment and cell-to-cell aggregation (Koczan et al., 2009). E. amylovora strains that do not have the capacity to produce amylovoran, are non-pathogenic, unable to spread in plant vessels (Bellemann and Geider, 1992) and die rapidly after host plant inoculation (Koczan et al., 2009). The adaptation of Podoviridae phages to amylovoran can, therefore, be considered an efficient survival strategy (Roach et al., 2013). In contrast to podoviruses, Myo- and Siphoviridae phages do not express EPS depolymerase activities in culture (Lehman et al., 2009), and at least the Myoviridae do not carry a gene encoding EPS depolymerase (Müller et al., 2011b). It is not known to date how these phages bypass the EPS barrier and infect their hosts (Roach et al., 2013).
During recent years, whole genome sequences have been determined for E. amylovora phages representing the Myo-, Sipho-, and Podoviridae (Lehman et al., 2009; Müller et al., 2011a; Born et al., 2011; Dömötör et al., 2012; Meczker et al., 2014). Many phage genomes, especially those of siphoviruses, appear as mosaics composed of genetic modules, i.e. single genes or groups of genes that are functionally exchangeable. These modules include head and tail and possibly further genes (Ackermann, 2011). It has, for instance, been found that crosses between the genomes of λ-like siphoviruses and P22-like podoviruses are able to form functional hybrids (Botstein and Herskowitz, 1974). In 2008, the lytic phage phiEcoM-GJ1 that attacks Escherichia coli of serotypes O149:H10:F4 has been described. According to its morphology comprising an icosahedral head, a neck and a long contractile tail with tail fibers, phiEcoM-GJ1 appears a typical myovirus, and expectedly shares significant similarities with E. amylovora phage Y2 (Myoviridae) throughout late gene cluster. However, phiEcoM-GJ1 carries an RNA polymerase encoding gene characteristic of T7 group podoviruses and several further genes encoding orthologs of siphovirus proteins (Jamalludeen et al., 2008).
In tailed bacteriophages, DNA packaging requires a terminase complex which consists of small and large subunits. The large terminase subunit is responsible for prohead binding, DNA translocation and DNA cleavage, while the small subunit is involved in DNA recognition and binding (Christie and Dokland, 2012; Feiss and Rao, 2012). According to the currently available E. amylovora phage genome sequences, all phages comprise a terminase large subunit (tls) gene, whereas a gene encoding terminase small subunit seems to be lacking in the Myoviridae. The present study reports on the use of a partial sequence of the tls gene as genetic marker for the preliminary PCR-based characterization of a set of E. amylovora bacteriophages isolated in the Republic of Moldova.
Research Design and Methodology
Samples for both E. amylovora and bacteriophage isolation were collected from apple orchards and Rosaceae plants growing in Kishinev municipality, Republic of Moldova (Table 1). Sampling sites are distributed over an area of 123 km². E. amylovora strain Ea179 was obtained from Prof. Klaus Geider (Julius Kühn-Institute at Dossenheim, Germany).
Phages were isolated and purified using standard procedures (Adams, 1959; Schnabel and Jones, 2001; Boulé et al., 2011). Aerial tissues and/or soil from beneath fire blight infected trees were mixed with sterile water. Samples were filtered through a 0.45 micron membrane filter and 5 ml of the phage filtrate used to inoculate 5 ml of E. amylovora broth cultures that were subsequently incubated at +28oC under agitation. After 18 h cultures were centrifuged at 4°C and 8,000 xg for 20 min. A mixture of 100 μL of the supernatant with 100 μL of E. amylovora hosts was added to 3 mL of soft agar (0,7%) and overlaid to hard agar (1,5%). Plates were incubated at +28oC. Lawns were routinely checked for plaque formation after 24 h and 48 h. Single plaques were picked, transferred to 1 mL nutrient broth containing 10 μL chloroform and centrifuged at 8,000 xg for 5 min. Resulting supernatants were transferred to sterile micro-centrifuge tubes and plated in triplicate using the soft agar overlay method. Phage preparations were diluted to obtain a titer corresponding to app. 50 phages per plate. Both E. amylovora strain Ea179 and further strains isolated in the Republic of Moldova were used as bacterial enrichment hosts. Bacteria and phages were cultured on nutrient agar (Obolensk, Russia) with fish meal pancreatic digest at 24 g/l H2O, NaCl 4.0 g/l, and agar 12.0 g/l, and incubated at 28°C. For liquid culture, a nutrient broth with enzymatic peptone digest at 100 g/l H2O, NaCl 50.0 g/l, KNO3 1.0 g/l, NaHCO3 10 g/l, and Na2S2O5 3.5 g/l was used.
The genomic DNA of phages was extracted and purified according to standard procedures using a DNeasy Blood & Tissue Kit (Qiagen, Germany). Optimized PCR reactions were run in a Biometra TGradient cycler under the following conditions: initial denaturation at 94°C for3 min; 35 three-step cycles at 94°C for 30 s, 50°C for 30 s, and 72°C for 3 min; final elongation at 72°C for 5 min. PCR reactions were analyzed by 1.0% horizontal agarose gel electrophoresis; ethidium bromide-stained bands were visualized under UV light. North American E. amylovora phage isolates phiEa1h (Podoviridae) and phiEa104 (Myoviridae) (Müller et al., 2011a) were used for specificity control reactions. Phylogenetic analysis of phage genome sequences was performed in MEGA5.2 (Tamura et al., 2011).
Results
A total of thirteen E. amylovora infecting phages were enriched and purified from environmental samples as specified in Table 1.
Table 1: Phage isolates investigated in this study
Based on the bacteriophage phylogeny reconstructed by Born et al., (2011) and using additional data from published phage genomes, sets of large terminase subunit genes of M7 group phages (i.e. more exactly the myoviruses phiEaM-M7 and phiWV8 as well as siphovirus psiM2) and L1 group phages (i.e. phiT7, phiYeO3-12, phiEaP-L1, phigh-1, phiSP6, and phiEra103 all belonging to the Podoviridae) were compared. Based on alignment results, degenerate primer pairs tlsM7F100/R500 and tlsL1F180/R550 (Table 2) were designed for the amplification of partial tls gene sequences from the genome of M7 and L1 group phages, respectively.
Table 2: Sequences of PCR primers used for marker amplification
When tls gene derived primer pairs were used in control reactions with the phage lysate from the L1 group podovirus phiEa1h, a PCR product of an apparent size of app. 1.1 kb was obtained with primer pair tlsL1F180/R550 as expected from the comparative analysis of tls gene sequences of L1 group phages. However, use of primer pair tlsM7F100/R500 with the same template DNA, while consistently lacking the app. 1.2 kb product expected for M7 phages, gave rise to two unsolicited PCR products considerably larger in size than 1.5 kb (Figure 1).
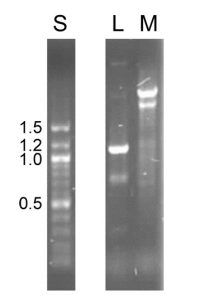
Figure 1: Control PCR from a phage phiEa1h lysate (Podoviridae, L1 group) using primer pairs tlsL1F180/tlsL1R550 (lane L) and tlsM7F100/tlsM7R500 (lane M);lane S: 100 bp ladder DNA size standard (NEB), sizes (in kb) are indicated at the left gel margin.
Application of primer pair tlsL1F180/tlsL1R550 to lysates of the Moldovan phage samples did not produce any signal (data not shown). In contrast, with primer pair tlsM7F100/tlsM7R500 several lysates yielded a PCR product of somewhat more than 1.2 kb expected for M7 group phages as did the myovirus phiEa104 lysate used as positive control (Figure 2).
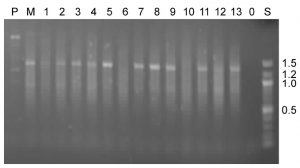
Figure 2: Diagnostic PCR using primers tlsM7F100/tlsM7R500. Lane P: phiEa1h (Podoviridae);lane M: phiEa104 (Myovridae); lanes 1-13: Moldovan phage isolates, numbering according to Table 1; lane 0: negative control; lane S: 100 bp ladder DNA size standard (NEB), PCR product sizes (in kb) are indicated at the right gel margin.
Discussion
Results obtained demonstrate that E. amylovora phages of groups L1 and M7 can be distinguished by the tls marker based approach developed. Application of this approach to phages sampled in the Republic of Moldova led to the positive identification of an M7 group virus in at least nine out of thirteen phage lysates. However, no L1 group phages were detected in this set of samples. While all soil derived phages were M7 positive, both M7 and L1 group phage identification failed for four out of nine samples from aerial parts of infected plants.
Throughout the sample set investigated, phage identification does not appear biased for the host plant. At the same time, it is known that the procedure of phage isolation has a great influence on the isolation of new bacteriophages. The nutritional status and the energetic state of the host cell, pH, temperature, and the availability of cofactors such as Ca 2+ or Mg 2+ can influence the efficiency of phage replication (Witzany, 2011). Moreover, the final product of a liquid enrichment culture will mostly be only the bacteriophage that is most competitive in this culture (Serwer et al., 2004).
As field derived E. amylovora strains from Moldova were used for phage isolation, the complete absence of L1 group phages might be a consequence of these bacteria being less susceptible to podoviruses as compared to M7 group phages due to, e.g., a low level EPS production. It is well documented that viral adaptation to a given host genotype often results in parallel evolution (Hall et al., 2013). Time-shift experiments over one season with a tree-bacteria-phage system using natural bacteriophages collected from the phyllosphere of horse chestnut trees provided clear evidence for both phage-mediated selection in bacterial and bacteria-mediated selection in phage communities in nature (Koskella, 2013). One possible outcome of such evolutionary dynamics can be regionally stabilized host-pathogens systems. For instance, Gill et al., (2003) reported that, with few exceptions, Podoviridae phages were unable to infect virulent E. amylovora bacteria from British Columbia. In the light if these findings it is tempting to speculate that a phage-bacteria system formed by virulent E. amylovora bacteria producing lower levels of amylovoran, and by M7 group bacteriophages adapted to these bacteria has stabilized in the investigated region. However, further phage isolation experiments using broad range enrichment strains have to be performed to rule out the possibility that the observed bias is a methodological artefact.
Müller et al., (2011b) have compared both Podoviridae and Myoviridae phages collected in Germany and North America and found that they differed considerably in genome size and molecular properties. For instance, genomic partial sequences recovered for German phage phiEaJ08C (Myoviridae) showed high and low similarity to the genomes of the American myoviruses phiEa116 and phiEa104, respectively. Moreover, the German Podoviridae phages phiEaJ08T and phiEaK08T gave rise to no or weak signals for primer pairs designed from the American podovirus phiEa1h. The results obtained in our experiments are consistent with a certain degree of heterogeneity among the set of phages collected in Moldova. In particular, four out of thirteen phages have not been positively identified as belonging neither to the M7 nor the L1 group.
Comparison of the fire blight control potential of bacteriophages belonging to the Podoviridae and Myoviridae families carried out by Müller et al., (2011b) on flowers and immature pears demonstrated that the podoviruses phiEa1h and phiEa100 had only a weak effect on growth reduction of E. amylovora in flowers reducing pathogen recovery by 40% as compared to controls; in contrast, the myoviruses phiEa104 and phiEa116 reduced pathogen recovery by 90%. For immature pears, however, no significant difference was observed between the biocontrol effects caused by Podoviridae and Myoviridae. Application of phage cocktails containing mixtures of Podoviridae and Myoviridae together with the pathogen for inoculation of apple flowers and pear slices did not significantly change symptom formation as compared to the application of a single myovirus. However, beyond assessment of the immediate control effect, application of complex phage cocktails might be a dominant strategy to prevent bacterial resistance development against viral biocontrol agents. A cocktail of siphoviruses phiEaH1 and phiEaH2 has successfully been employed in the control of E. amylovora (Meczker et al., 2014). In the light of these results, several Moldovan bacteriophage strains have been identified in this study as belonging the M7 group of viruses with elevated a priori biocontrol potential.
Targeted selection of bacteriophage strains that are efficient under field conditions may help to develop biological fire blight control agents. Born et al., (2013) presume that application of depolymerase enzyme of the Podoviridae phage L1 together with the broad host range, virulent Myoviridae phage Y2 represents an attractive combination for biocontrol of fire blight. An important consideration is the selection of phages that are better adapted to the relevant environmental conditions (Jones et al., 2007). The prevention of the blossom blight phase of the disease is a key point of fire blight management (Johnson and Stockwell, 1998; Norelli et al., 2003). If E. amylovora-specific phages are present on the blossoms, they might suppress the growth of E. amylovora on the stigmatic surfaces (Schnabel and Jones, 2001). From this point of view, a reasonable approach to targeted selection of E. amylovora phage strains for fire blight control would be their collection in the phyllosphere. In our study nine out of thirteen E. amylovora phages were isolated from aerial parts of the infected host plant and only 4 from soil surrounding the plants; all four phages from soil and five out of nine from the phyllosphere were identified as M7 group viruses.
In conclusion, despite needing further refinement and improvement on the basis of more available phage genome sequence data, the tls gene based diagnostic approach presented here positively identified nine out of thirteen E. amylovora phages isolated from around Chisinau, Republic of Moldova, as members of the M7 group of viruses. This group comprises bacteriophages of both the taxonomic families Myoviridae and Siphoviridae that are presumably more suitable for the development of efficient fire blight biocontrol agents. In contrast, no L1 group phages were identified, and four phage samples could not be assigned to any group of viruses. Thorough assessment of the biodiversity present among phage samples from Moldova will need further research efforts. Moreover, careful study of the bacteriophage–host interactions in the plant environment will be a prerequisite for the elaboration of both safe and efficient techniques for the application of bacteriophages in fire blight management.
Acknowledgment
We are highly indebted to Prof. Klaus Geider (Julius Kühn-Institute at Dossenheim, Germany) for providing E. amylovora strain Ea179 and phage isolates phiEa1h and phiEa104. This research work has been financially supported, including a scholarship to A.V. Samoilova, by grant 01DK12005 from the German Federal Ministry of Education and Research (BMBF).
References
- Ackermann, H. W. (2011) ”Bacteriophage taxonomy,” Microbiology Australia, 5 (May) 90-94.
- Ackermann, H. W. and Prangishvili, D. (2012) ”Prokaryote viruses studied by electron microscopy,” Archives of Virology, 157 (10) 1843-1849.
- Adams, M. H. (1959) Bacteriophages, Interscience Publisher, New York, N.Y. Translation into Russian (1961) Izdatelistvo Inostrannoi Literatury, Moscow.
- Bellemann, P. and Geider, K. (1992) “Localization of transposon insertions in pathogenicity mutants of Erwinia amylovora and their biochemical characterization,” Journal of General Microbiology, 138, 931-940.
- Bogs, J. and Geider, K. (2000) “Molecular analysis of sucrose metabolism of Erwinia amylovora and influence on bacterial virulence,” Journal of Bacteriology, 182 (19) 5351-5358.
- Born, Y., Fieseler, L., Klumpp, J., Eugster, M. R., Zurfluh, K., Duffy, B. and Loessner, M. J. (2013) The tail-associated depolymerase of Erwinia amylovora phage L1 mediates host cell adsorption and enzymatic capsule removal, which can enhance infection by other phage. [ Online]. Environmental Microbiology. [accessed August 14, 2013]. Available: http://www.ncbi.nlm.nih.gov/pubmed/23944160.
- Born, Y., Fieseler, L., Marazzi, J., Lurz, R., Duffy, B. and Loessner, M. J. (2011) “Novel virulent and broad-host-range Erwinia amylovora bacteriophages reveal a high degree of mosaicism and a relationship to Enterobacteriaceae phages,” Applied and Environmental Microbiology, 77 (17) 5945-5954.
- Botstein, D. and Herskowitz, I. (1974) “Properties of hybrids between Salmonella phage P22 and coliphage lambda,” Nature, 251 (5476) 584-589.
- Boulé, J., Sholberg, P. L., Lehman, S. M., O’gorman, D. T. and Svircev, A. M. (2011) “Isolation and characterization of eight bacteriophages infecting Erwinia amylovora and their potential as biological control agents in British Columbia, Canada,” Canadian Journal of Plant Pathology, 33 (3) 308-317.
- Christie, G. E. and Dokland, T. (2012) “Pirates of the Caudovirales,” Virology, 434 (2) 210–221.
- Dömötör, D., Becságh, P., Rákhely, G., Schneider, G. and Kovácsa, T. (2012) “Complete genomic sequence of Erwinia amylovora phage PhiEaH2,” Journal of Virology, 86 (19) 10899.
- Feiss, M. and Rao, V. B. (2012) “The bacteriophage DNA packaging machine,” Advances in Experimental Medicine and Biology, (726) 489-509.
- Gill, J .J., Svircev, A. M., Smith, R. and Castle, A. J. (2003) “Bacteriophages of Erwinia amylovora,” Applied and Environmental Microbiology, 69, 2133-2138.
- Hall, J. P., Harrison, E. and Brockhurst, M. A. (2013) “Viral host-adaptation: insights from evolution experiments with phages,” Current Opinion in Virology, 3, 572-577.
- Jamalludeen, N., Kropinski, A. M., Johnson, R. P., Lingohr, E., Harel, J. and Gyles, C. L. (2008) “Complete genomic sequence of bacteriophage ϕEcoM-GJ1, a novel phage that has Myovirus morphology and a Podovirus-like RNA polymerase,” Applied and Environmental Microbiology, 74 (2) 516–525.
- Johnson, K. B. and Stockwell, V. O. (1998) “Management of fire blight: a case study in microbial ecology,” Annual Review of Phytopathology, 36, 227-248.
- Jones, J. B., Jackson, L. E., Balogh, B., Obradovich, A., Iriarte, F. B. and Momol, M. T. (2007) “Bacteriophages for plant disease control,” Annual Review of Phytopathology, 45, 245-262.
- Koczan, J. M., McGrath, M. J., Zhao, Y. and Sundin, G.W. (2009) “Contribution of Erwinia amylovora exopolysaccharides amylovoran and levan to biofilm formation: implications in pathogenicity,” Phytopathology, 99 (11) 1237-1244.
- Koskella, B. (2013) “Phage-mediated selection on microbiota of a long-lived host,” Current Biology, 23 (13) 1256-1260.
- Lehman, S. M., Kropinski, A. M., Castle, A. J. and Svircev, A. M. (2009) “Complete genome of the broad-host-range Erwinia amylovora phage ΦEa21-4 and its relationship to Salmonella phage Felix O1,” Applied and Environmental Microbiology, 75 (7) 2139–2147.
- Meczker, K., Dömötör, D., Vass, J., Rákhely, G., Schneider, G. and Kovács, T. (2014) “The genome of the Erwinia amylovora phage PhiEaH1 reveals greater diversity and broadens the applicability of phages for the treatment of fire blight,” FEMS Microbiology Letters, 350 (1) 25-27.
- Müller, I., Kube, M., Reinhardt, R., Jelkmann, W. and Geider, K. (2011a) “Complete genome sequences of three Erwinia amylovora phages isolated in North America and a bacteriophage induced from an Erwinia tasmaniensis strain,” Journal of Bacteriology, 193 (3) 795–796.
- Müller, I., Lurz, R., Kube, M., Quedenau, C., Jelkmann, W. and Geider, K. (2011b) “Molecular and physiological properties of bacteriophages from North America and Germany affecting the fire blight pathogen Erwinia amylovora,” Microbial Biotechnology, 4 (6) 735–745.
- Norelli, J. L., Jones, A. L. and Aldwinckle, H. S. (2003) “Fire blight management in the twenty-first century using new technologies that enhance host resistance in apple,” Plant Disease, 87, 756-765.
- Roach, D. R., Sjaarda, D. R., Castle, A. J. and Svircev, A. M. (2013) “Host exopolysaccharide quantity and composition impact Erwinia amylovora bacteriophage pathogenesis,” Applied and Environmental Microbiology, 79 (10) 3249–3256.
- Samoilova, A. V. and Terteak D. D. (2012) “Bacteriophages of Erwinia amylovora, perspective in the fire blight control,” Conference Proceedings of IOBS Plant Protection: Problems and Perspectives, (2012), 242-245.
- Schnabel, E. L. and Jones, A. L. (2001) “Isolation and characterization of five Erwinia amylovora bacteriophages and assessment of phage resistance in strains,” Applied and Environmental Microbiology, 67 (1) 59-64.
- Serwer, P., Hayes, S. J., Zaman, S., Lieman, K., Rolando, M. and Hardies, S. C. (2004) “Improved isolation of undersampled bacteriophages: finding of distant terminase genes,” Virology, (329) 412–424.
- Tamura, K., Peterson, D., Peterson, N., Stecher, G., Nei, M. and Kumar, S. (2011) “MEGA5: Molecular evolutionary genetics analysis using maximum likelihood, evolutionary distance, and maximum parsimony methods,” Molecular Biology and Evolution, (28) 2731-2739.
- Witzany, G. (2011) Biocommunication in Soil Microorganisms, Springer-Verlag, Heidelberg.