Introduction
Water pollution is owing to all human activities including domestic, industrial and agricultural and thus, the causes of pollution in water can be considered endless. However, manufacturing plants is a major cause of water pollution that carries away their waste that can contain various dissolved or suspended harmful and toxic substances in fresh water bodies. Suspended sub-stances, nutrients and organic load as chemical oxygen demand (COD) contribute major pollutants in these bodies. Therefore, the removal of these contaminants in waste water is one of the fundamental targets in waste treatment [1]. Further, stringent regulations are releasing in recent years to prevent discharge of these wastes into the municipal sewerage system without any treatment.. Many technologies are developed to treat various types of wastes at adequate levels. However, the economic performance, including investment costs and operation of conventional waste treatment are prohibitive for a variety of industrial simulation, particularly small-scale sector. In this case, the physical-chemical coagulation-flocculation process is a most used key step in the primary purification of industrial wastewater and effluents and in some cases in secondary and tertiary treatment [2].
Generally, such solid-liquid separation processes are carried out by using inorganic or organic coagulating agents. The most common extensively used inorganic coagulants are alum and iron salts. The multivalent characteristics of these cations strongly attract to the colloidal particles rendering them insoluble. On the other hand, organic coagulating agents are also widely used such as polyelectrolyte (cationic or anionic), polyampholytes and nonionic polymers are more efficient agents than their inorganic counterparts and thus, they don’t produce voluminous and gelatinous flocs [3]. Surface-adsorbed cationic and anionic polyelectrolytes or polyampholytes promote suspended particle coagulation rates by two distinct mechanisms: charge neutralization and/or polymer bridging [4-5].
Although the synthetic polymers can be tailored to the needs of a particular application and perform well in flocculation, they are often associated with environmental pollution. Natural polymers have the advantages of low cost and biodegradability, but the biodegradability will affect the storage life and the flocculation performance. Therefore, several attempts have been made to combine the best properties of both by grafting synthetic polymers onto the backbone of natural polymers [6]. One of the advantages gained is the consequent reduced biodegradability because of a drastic change in the original regular structure of the natural polymer as well as the increased non-degradable synthetic polymer content within the product. However, the grafting of shear degradable polymers onto a rigid polysaccharide backbone was found to produce fairly shear-stable systems [8-9].
Chitosan is a cationic linear pseudo-natural polymer obtained from N-deacetylation of chitin in alkaline media. Chitosan has several intrinsic properties such as biocompatibility, biodegrada-bility and its degradation products are non-toxic, as well as, its outstanding chelating behavior and thus it directed to numerous applications in waste water treatment, chemical industry, pharmacy, biotechnology and biomedicine [9-13]. Chitin and chitosan are widely used for waste water treatment for the removal both of dispersed particulates and dissolved pollutants. Further, water treated with these polymers experimentally showed a decrease in COD, total nitrogen and microbial population [14-15]. However, acidic solubility, low thermal and mechanical stability and flexible behavior of chitosan are the stumbling blocks in broadening its application in water treatment. Many physical and chemical modification approaches have been developed to jump over these blocks. Recently, a lot of attention has been paid to chemical modification. Though many researchers have shown that primary derivatization followed by graft copolymerization was one of the promising methods to improve tremendously chitosan properties, particularly, via amalgamation of synthetic polymers [16-21]. Grafting flexible polyacrylamide chains onto rigid polysaccharides such as chitosan has been suggested to have a potential to develop efficient, shear-stable, and controlled biodegradable flocculants for the treatment of industrial and mineral processing effluents [22]. In the present study, carboxymethyl chitosan (CMCH) was chosen as polysaccharide backbone with polyacrylamide as a graft polymer for the preparation of amphoteric flocculants. Whilst chitosan (CH) is a cationic polyelectrolyte, carboxyalkyl chitosan can be considered a polyampholyte which carry positive and negative charges [23]. Thus, the synthesis of graft copolymer was carried out using PPS-induced free radical polymerization. The effect of concentration of CMCH, AM and PPS as well as the reaction temperature and time on the graft copolymerization was studied by determining the grafting parameters. Moreover, the flocculation performance of the obtained grafted copolymers was investigated by studying the effects of pH values, flocculation time and dosage of the flocculants on treating kaolinite suspensions.
Materials and Methods
Materials
Chitosan (DD=85%), potassium persulphate (PPS), chloroacetic acid, acetic acid (99%), acry-lamide (monomer), and glutaraldehyde (60%) were obtained from Sigma-Aldrich chemical LTD (Germany). Acetone (99.9%), sodium hydroxide, was purchased from El-Nasr pharmaceutical company for chemicals (Egypt). Kaolinite (Egyptian highly refined ball clay powder with very fine size) used mainly for manufacturing refractory bricks and mortar, was kindly donated from Alexandria Company for Refractories.
Preparation of carboxymethyl chitosan (CMCH)
Carboxymethyl chitosan was prepared according to the method reported by Chen and Park [24]. Briefly 10 g of chitosan were dispersed in 100 ml of aqueous isopropyl alcohol (50 %) with stirring at room temperature for 30 min. Aliquot portions of 15 ml sodium hydroxide (10 M) were added at an interval of 5 min under agitation at room temperature. The reaction mixture was stirred for an additional 45 min. Then, 30 g of chloroacetic acid was dissolved in this mixture portion wise at interval of 5 min under warming at 55-60 °C with stirring for 1 h. The resultant solution was filtered. The filtrate was washed with aqueous solution of ethanol (80 % v/v), then dried in a vacuum oven at 50 °C for 24 hrs.
Preparation of carboxymethyl chitosan polyacrylamide graft copolymers (CMCH-g-PAM)
Definite amounts (0.3 -24 gm) of CMCH were dissolved in 45 ml distilled water, then (0.05 — 1 gm) PPS dissolved in 10 ml distilled water then slowly added to the three necked flask to initiate graft copolymerization. In a constant temperature, water bath was maintained at a constant temperature in the range of 25 – 60 â°C. Nitrogen gas was bubbled for 30 min to remove the dissolved oxygen under stirring. After 10 min, a predetermined amount (1 – 6 gm) of acrylamide dissolved in 45 ml distilled water was charged into a three necked round bottom flask. After grafting time (1-5 hrs), CMCH-g-PAM was precipitated by the addition of an excess of acetone (80 %), ï¬ltered, and then the precipitate was washed by aqueous ethanol solution (90% v/v) to elimi-nate all of CMCH, AM and its homopolymer. Afterwards, graft copolymers were dried under vacuum at 50 â°C. The grafting percentage was calculated using the following equation;
% G = [ WCMCH-g-PAM / WCMCH] x 100 % [25]
FTIR spectroscopic analysis
FTIR spectra were obtained by a Shimadzu AIM8400 FTIR spectrometer equipped with ATR-8400M objective (Shimadzu Co, Ltd, Tokyo, Japan). This objective adopted a germanium prism tip. The spectra were recorded over a range of 500-4000 cm-1 with a resolution of 2 cm-1 and averaged over 32 scans.
Thermogravimetric analysis (TGA)
Thermal analysis was performed by using Shimadzu TGA-50 Analyzer (Shimadzu Co. Ltd., Kyoto, Japan). Specimen (10 mg) were thermally scanned from 25 to 900°C in the open corundum crucibles under nitrogen with a flow rate of ≈ 200 ml/min) at a scanning rate 10° C/min.
Scanning Electron Microscopy (SEM)
Microstructure and topography of CMCH and CMCH-g-PAM films were characterized by a JEOL JSM-6360 LA scanning electron microscope (JEOL Ltd., Rigaku, Tokyo, Japan). Examination was performed at an acceleration voltage of 15 kV with a magnification power of 1000-3000X. Film samples were first coated with gold using JFC-1100E sputtering coater.
Swelling measurement
Definite weights (2 gm) of CH, CMCH or CMCH-g-PAM were put onto sintered glass funnel, im-mersed in 1000 ml beaker filled with distilled water and then covered with Para-film. Afterwards, water was withdrawn by water pump throughout constant time of 15 min, and then weight the swollen samples. Weight values were taken at equilibrium, which was determined by three consecutive measurements having the same weight. The swelling ratio of the specimens was calculated according to the following equation:
SR= Ws – Wd / Wd [26]
Where Ws and Wd represented the weights of the swollen and dry state samples, respectively. The dynamic weight gain was determined versus time to illustrate the swelling behavior of these polymers.
Flocculants performance assessment (Jar Test)
To prepare the clay suspension (turbid water), accurate weighed amounts (5 – 20 gm) of kaolinite powder were dispersed in distilled water with stirring using mixer at 500 rpm for 2 min. Adjusting pH value (4-9) during stirring was achieved using 0.1N NaOH or HCL. Then stirring continues for another 2 min at a rate of 100 rpm. Temperature keeps in water bath at constant degree, with adding NaCl to obtain solution with ionic strength ranging from 0.1 to 0.6 M. Af-terwards, allow the dispersed solutions to settle for 1 – 20 min., before sampling using automatic pipette from a height of 2 cm below the surface in each beaker for turbidity measurement. For assessing flocculants’ efficiency, control (without flocculants) was first used to measure the initial turbidity (To), and so, the turbidity of the clay suspension after adding different amounts of the flocculants (1 — 10 ppm) (Tt) was measured using the same sampling method. The turbidity removal percent was calculated by the following equation;
Turbidity removal % = To-Tt / To [27]
Results and Discussion
Factors affecting grafting percentage
CMCH concentration
Generally, grafting percentage (%G) was found to increase significantly with increasing CMCH concentration as shown in Figure 1, where %G was 66 at CMCH concentration of 0.3 %, however it reached its maximum (146 %) at CMCH concentration of 2 %. Whereas, further increase in CMCH concentration led to a significant decrease in grafting percentage which reached to 18% at CMCH concentration of 24 %. The initial increase in the grafting percentage associated with the increase of carboxymethyl chitosan concentration can be attributed to the increase in the number of active sites (amine and hydroxyl groups) on carboxymethyl chitosan molecules leading to accommodate higher amounts of acrylamide monomer (AM) and consequently increase the produced grafted PAM (scheme 1). Whereas, further increase in CMCH concentration may increase the viscosity of the reaction medium that can cause hindrance of the reactants’ collision and thus reducing their reactivity, in addition to a decrease of AM / CMCH ratio. These findings were in agreement with those obtained in the study of Ranjbar-Mohammadi with his colleagues on grafting chitosan onto wool fabric [26].
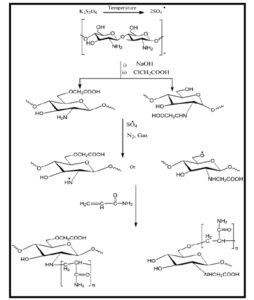
Scheme 1: CMCH grafting mechanism
Figure 1: Effect of CMCH concentration on grafting percentage
Monomer concentration
The grafting percentage grew dramatically with increasing AM concentration reaching its maximum value at AM concentration of 6 % (Figure 2). However, higher increase in AM con-centration led to a noticeable decrease of the grafting percentage. These results were not in agreement with the results reported by Ãvila with his workers [7]. This behavior could be ex-plained by the fact that an increase of AM concentration leads to the accumulation of monomer molecules in close to the CMCH backbone. Hence, increasing monomer concentrations over sa-turation could be in favor of free radical-induced polymerization of AM monomer on expense of its reaction with CMCH polymer backbone. i.e., homopolymerization overlies on grafting copo-lymerization. Furthermore, the formation of homopolymer in the presence of an excess of mo-nomer can shield the polymer backbone and consequently stands as a hurdle towards graft co-polymerization. Such assumptions can be associated with diffusion limitations, which can be governed by the dilution [26].
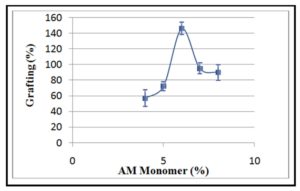
Figure 2: Effect of monomer concentration on grafting percentage
Initiator concentration
Indeed, initiator concentration is well known to have a crucial impact on polymerization. Therefore, determining the optimum concentration of PPS in view of the enhancement of grafting was achieved by using concentrations in the range of 0.05 — 1 % at constant concentrations for both CMCH and AM. The obtained results were presented in Figure 3. These results indicated that the grafting percentage increased with increasing the initiator concentration up to 0.1 %, thereafter it decreased gradually with the further increase in PPS concentration. The increase of grafting percentage can be attributed to the increase of active grafting sites on the CMCH backbone chains (free radicals) with the increase of initiator concentration which in turn led to higher grafting. While, its decrease at PPS concentration over 0.1 % can be explained on the basis that excessive free radicals produced simultaneously at these high concentrations could activate multiple free radicals coupling that led to the formation of AM homopolymer or cross-linking CMCH since free radicals formed onto CMCH chains can be terminated prior to AM addition [28]. Hence, the formation of homopolymers can be expected due to the non-availability of active sites on CMCH. On the other hand, at higher initiator concentration, PPS can decompose to HSO4- and O2 whilst O2 act as scavenger for free radical formed on polymer backbone chains and thus decreasing the grafting polymerization [29].
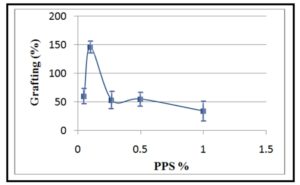
Figure 3: Effect of initiator concentration on grafting percentage
Grafting temperature
As shown from Figure 4 when grafting copolymerization was accomplished at varying temperature in the range of 45 – 65°C, highest grafting percentage was attained at 55°C, while it was decreased at lesser or upper optimum temperature. These findings can be explained in view of the fact that the low temperatures are insufficient to activate free radicals’ formation. However, obtaining the highest grafting percentage at optimum temperature can be ascribed to its ability to promote formation of free radicals and swelling of CMCH leading to enhance diffusion of AM monomer. The further increase in temperature can be in favor of the combination of macro-radicals to be dominant reactions leading to the formation of AM homopolymer which increases the viscosity of the reaction medium and thereby hinders for the diffusion of monomer and inhibits graft copolymerization [30]. Based on the aforementioned, the optimum temperature meets the halfway between both tendencies, since it facilitates the diffusion of AM monomer into CMCH phase and activates the formation of free radicals via increasing the dissociation rate of initiator to be in the active form [29].
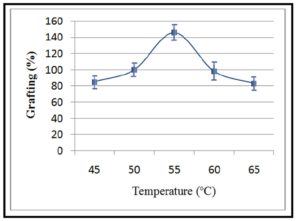
Figure 4: Effect of temperature on grafting percentage
Grafting time
The effect of the reaction time on the grafting percentage was presented in Figure 5. The grafting percentage was gradually increased with extending the reaction time from 1 to 3 hrs. Nevertheless, it decreased with the prolongation of the reaction time over 3 hrs. These findings are in agreement with those of Joshi and Sinha that reported that there is a slight decrease in the grafting percentage with the increasing time of polymerization, and thereafter it becomes constant [22]. The increase of the grafting percentage with the prolonging time of the reaction can be attributed to give a break to produce more free radicals onto polymer backbone and open up its structure. Forasmuch, the bulky groups, such as carboxmethyl (ï€ CH2COOH) in the CMCH can promote the swelling and thereby diffusion of PPS and AM into CMCH [30]. However, the observed decrease in the grafting percentage with the long time of reaction can be explained on the basis of depleting acrylamide monomer with the progression of the grafting process. Furthermore, this decrease may be also attributed to enhance the viscosity of the reaction medium by growing the yield of graft copolymers and subsequently slow down reactants’ collision and lower grafting ratios [29].
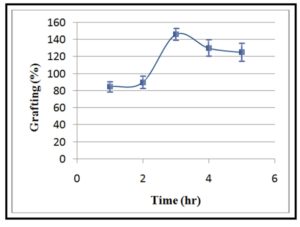
Figure 5: Effect of grafting time on grafting percentage
Physico-chemical properties characterization
Compositional properties
The spectra of CH and grafted polymer (CMCH-g-PAM) were shown in Figure 6. FTIR spectrum of chitosan exhibited two strong characteristic absorptions peaks; one ~1575 cm−1 due to N—H bending and other peak assigned to Nï€H and Oï€H stretching at ~ 3369 cm-1. Furthermore, ad-sorption peak appeared at 1321 cm-1 due to Cï€N bending, in addition to a small band at 1649 cm-1 which refers to (C=O) of acetamide group (-CONH-) belonging to acetylated unites of chitosan. However, FTIR spectrum of CMCH-g-PAM exhibited an absorption band at ~ 1662 cm-1 due to C=O (amide) stretching vibrations, peak at ~1423 cm-1 of COO – stretching vibrations and peak at ~2945 cm-1 due to the Cï€H of CH2 stretching vibrations. Such graft copolymer spectrum also showed more intense broad adsorption peak appeared at ~ 3390 cm-1 due to the overlapping of O—H and N—H stretching of CMCH and PAM. The slight shifting and more intensity of the latter absorption peak confirm grafting reaction between carboxymethyl chitosan and polyacrylamide.

Figure 6: FTIR spectra of Chitosan (A) and CMCH-g-PAM copolymer (B)
Thermal stability
Thermal stability of CH and CMCH-g-PAM were assessed by thermogravimetric analysis. Figure 7 presented the thermograms of chitosan and graft copolymer. The thermogram of chitosan exhibited four distinct stages of thermal degradation; weight loss 13.8 % in the range of 27 ï€152 â°C was corresponding to the loss of adsorbed and bound water. Since chitosan contains (NH2) and (OH) functional groups, the hydrogen bonding force is strongly formed among molecules [31]. The second stage of the thermal degradation occurred at 220 – 455 â°C with weight loss was ~ 21 %. This weight loss can be attributed to degradation and deacetylation of chitosan [32]. Further weight loss by ~ 20 % occurred at temperature range 455 ï€ 800 â°C due to the dehydration of glycoside unit and decomposition of the acetylated and deacetylated units of chitosan. On the other side, CMCH-g-PAM exhibited also four subsequent distinct degradation (weight loss) stages (25 ï€ 799 â°C) ; first stage was from 25 ï€ 168 â°C with weight loss ~ 16 % due to the loss of adsorbed and bound water, second stage 228 ï€ 352 â°C during which ~ 17 % weight loss was assigned to decarboxylation of CMCH and subsequent loss of CO2. Afterwards, ~26 % weight loss between 354 ï€ 472 â°C that contributes to the degradation of both the CMCH backbone and the grafted polyacrylamide chains amalgamated onto CMCH-g-PAM. Finally ~22 % weight loss up to 799 â°C due to the decomposition of the glycosidic units of CMCH. These findings revealed that grafting slightly increased the initial decomposition temperature of CMCH-g-PAM compared with that for chitosan.
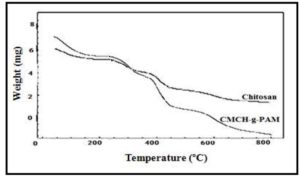
Figure 7: Thermograms of Chitosan and CMCH-g-PAM copolymer
Morphological and micro-structure properties
Scanning electron micrographs of carboxymethyl chitosan and its graft copolymer with PAM were shown in Figure 8. Figure 8A exhibited cohesive and dense structure of CMCH via appearing relatively smooth and continuous surface with no distinct perturbations. Whereas, SEM micrograph of CMCH-g-PAM (Figure 8B) showed that the graft copolymerization of chitosan with polyacrylamide dramatically changed the conformation of CMCH polymer chains, since the heterogonous structure was observed because the interruption of the chain packing occurs by conformational defects leading to major disruption in the perfection of the chain packing. These conformational defects in CMCH chains can be attributed to the grafted PAM chains and conse-quently confirms graft polymerization.
Figure 8: Scanning electron micrographs (SEM) of CMCH (A) and CMCH-g-PAM co-polymer (B)
Swelling of CH, CMCH and CMCH-g-PAM was gravimetrically determined by measuring the weight gain with time when they were placed in distilled water at room temperature. Measurements were taken at equilibrium, which was determined by three consecutive measurements having the same weight. Chitosan and CMCH gave swelling by 40 and ~ 50 %, respectively at 15 min, after which these polymers were going to dissolve. However, swelling of grafted copolymer reached to ~55% at 30 min. (Figure 9). Change swelling behavior of chitosan may be attributed to the introduction of carboxymethyl groups that improve remarkably the solubility of CMCH in aqueous solution [33]. However, hydrogen bonding is responsible for enhancing moisture retention ability of graft copolymer, where, the hydrophilic groups such as carboxylic and amino groups is known to form Hï€bonds with water molecules.
Figure 9: Swelling percentages of chitosan, CMCH and CMCH-g-PAM vs. time
Factors affecting flocculation (turbidity removal of clay suspensions)
Polymer type
The compositional characteristics of the polymer-based flocculants including both type and content of functional groups, branching, and molecular weight of the polymer are considered to be among the main factors affecting the flocculation efficiency. Therefore, the flocculation per-formance of CH, CMCH, PAM, and CMCH-g-PAM was investigated by measuring the turbidity of kaolinite suspensions after flocculation (Figure 10). From these results, it was observed that CMCH-g-PAM grafted copolymer has the highest flocculation efficiency. On the other hand, chi-tosan revealed the lowest efficiency. However, the efficiency of CMCH was comparable to that of PAM. High flocculation performance of graft copolymer can be due to their loose and branched structures giving potential flexible behavior that enhances the bridging effect of these flocculants. Additionally, charge density arising from various charged functional groups (-COO, -NH2) on these flocculants provides flocculation power towards fine dispersed kaolinite particulates. This was on contrary of CH, CMCH, and PAM which are considered linear polymers.
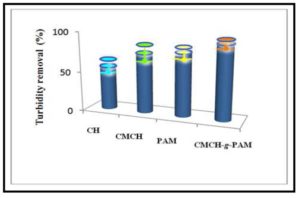
Figure 10: Turbidity removal of clay suspension using chitosan, CMCH, PAM and CMCH-g-PAM
pH
In the present study, the initial turbidity of kaolinite suspensions (turbid water) was measured at different pH values. These measurements indicated that the initial turbidity was increased by increasing pH as presented in Figure 11. This can be due to the increase of — ve charge density on kaolinite particulates in alkaline media (at basic pH 9) that increase repulsion between clay particles together and with —ve hydroxyl groups of basic medium which prevent aggregation of the particles. On the contrary, the highest percentages of turbidity removal from clay suspensions using CH, CMCH and CMCH-g-PAM were observed in acidic medium (pH = 6) (Figure 12). This result can be attributed to increasing + ve charge density on CH, PAM, CMCH and CMCH-g-PAM as a result to protonation of amine groups that form + ve charge centres on these polymer molecules, whereas, kaolinite particles have — ve zeta potential in this acidic medium and consecutively so strong electrostatic attraction has been achieved between oppositely charged macromolecular flocculants and clay particles (Charge neutralization) . However, the superior flocculating efficiency of CMCH-g-PAM and CMCH compared with CH can refer to higher charge density arising from many of amine groups carrying a positive charge at the acidic pH and thus the hydrophilicity and wettability of these polymer molecules were increased. Furthermore, high molecular weight and branching of graft copolymer enhance the flocculation performance since the extended chain tends to be adsorbed onto the particle in a flat configuration, whereas the negative charges on the bare parts of surface of one particulate easily attach to the excess net residual positive charges of the polymer absorbed on another one [34, 35]. On the other side, CMCH-g-PAM accomplished only about 75 % of turbidity removal at neutral pH as shown in Figure 12. Such lower efficiency of turbidity removal occurring at pH 7 compared with that occurring at acidic pH can be attributed to that at this neutral pH, kaolinite zeta potential becomes more — ve, whilst less protonation of amine groups leads to lower + ve zeta potential of flocculants than that occurring in acidic medium and consequently attraction force between clay particulates and polymer can be reduced. In alka-line medium (pH = 9), the turbidity removal percentage of grafted copolymer decreased to about 37 %. This can be due to — ve zeta potentials for both carboxylate groups of graft copolymers and kaolinite particulates and simultaneously less protonation of amine groups, therefore the resultant electrostatic repulsion can reduce the flocculating performance [6,36].
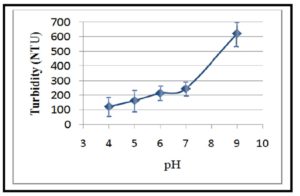
Figure 11: Effect of pH on the initial turbidity of clay suspension (10 gm/L)
Figure 12: Effect of pH of clay suspension (10 gm/L) on turbidity removal using flocculants at dosage of 5 ppm
Grafting percentage
The grafting percentage of CMCH-g-PAM exhibited a noticeable effect on the flocculation efficiency (Figure 13). In general, turbidity removal percentage for clay suspension using graft copolymer was noticeably increased with increasing the grafting percentage, so the graft copolymer having the highest grafting percentage (146%) exhibited efficiency of the turbidity removal that reached ~ 90 % with a noticeable mechanical stability for the resultant flocs (Figure 14). Since these expanded polymer chains play an axial role in bridging mechanisms that are highly responsible for flocculation performance. These results can be explained on the basis that increasing grafting ratio was associated with increasing the molecular weight of polymers and the length of the grafted polymer chains that led to enhancing flocculating performance via bridging mechanism. On the other hand, positive charges of protonated amine groups (NH3+) mounted onto PAM long branched chains linked to the CMCH backbone, whilst these CMCH backbone chains carry negative charge on anionic carboxylate groups (— COO¯ ) when dissociate at pH 6 enhancing the flocculation performance via charge neutralization mechanism. Hence, the adjacent charged groups that repel each other will lead to expanding the polymer chains. Therefore, the bridging by high molecular weight polymer chains can be considered the main mechanism in the flocculation of kaolinite particulates with the contribution of role of ionic functional groups in charge of neutralization, because increasing the degree of ionicity (charge density) of the more expanded form of polymer chains leads to enhancing flocculating power with increasing the rate of settling, i.e., increasing of the floc size [37].
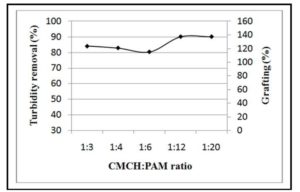
Figure 13: Effect of grafting percentage on turbidity removal from clay suspension (10 gm/L) at pH 6 using CMCH-g-PAM at dosage of 5 ppm
Figure 14: Photograph indicating turbidity removal from kaolinite suspension (10 g/L) using chitosan and CMCH-g-PAM (% G = 146), at dosage of 2.5 ppm and pH 6
Conclusion
In the present study, carboxymethyl chitosan was successfully grafted with acrylamide using potassium persulphate as an initiator. Graft copolymers with high grafting percentages reached to 146% were obtained. The optimization of the parameters reaction were studied. The finding of these experiments indicated that performing grafting copolymerization using carboxymethyl chitosan and acrylamide at the concentration of 2 and 6%, respectively in the presence of 0.1% of initiator at 55ºC for 3 hrs resulted in graft copolymer having the highest grafting percentage. The graft copolymers were structurally characterized using FTIR, and SEM. Moreover, the thermal stability of the graft copolymer was assessed using TGA. These analyses confirmed the formation of graft copolymer having higher initial thermal decomposition temperature. The flocculation performance of the obtained graft copolymer was tested using kaolinite suspensions, where the effect of grafting percentage and dosages of graft copolymer and pH on flocculation performance were studied with comparison to chitosan, CMCH and PAM. Under the same conditions, CMCH-g-PAM was found to be more efficient than chitosan, carboxymethyl chitosan and po-lyacrylamide.
Acknowledgements
The authors highly appreciate that this work has been funded by Egyptian Academy of Scientific Research and Technology (ASRT).
References
1. Shamim, A, Kaneco, S, Ohta, K, Mizuno, T. and Kani, K. (2001)” Use of some natural and waste materials for waste water treatment,” Water Research, 35 (15) 3738—3742.
Publisher – Google Scholar
2. Edeline F. (1992) L’épuration Physico-chimique des eaux :Théorie et technologie, Editions Cebedoc (éditeur), Lavoisier Technique et Documentation, 2e édition, liege, Paris.
3. Menoret C. (2001) Thèse du Doctorat en sciences de l’eau et de l’environnement continental, Université MONPELLIER II, France, 116.
4. Gregory, J. (1973) “Rates of flocculation of latex particles by cationic polymers,” Journal of Colloid and Interface Science, 42 (2) 448-456.
Publisher – Google Scholar
5. Bottero, J.Y. D, Tchoubar, M. A. V, Quienne, A. P. and Fiessinger, F. (1990) “Flocculation of silica colloids with hydroxy aluminum polycations: Relation between floc structure and aggre-gation mechanisms, Langmuir, 6 (3) 596-602.
Publisher – Google Scholar
6. Sang, Y. and Xiao, H. (2008) “Clay flocculation improved by cationic poly (vinyl alco-hol)/anionic polymer dual-component system,” Journal of Colloid and Interface Science, 326 (2) 420—425.
Publisher – Google Scholar
7. Ãvila, A., Pucci, G., Bierbrauer, K., López-González, M. and Strumia, M. (2012) “Study of op-timization of the synthesis and properties of biocomposite films based on grafted chitosan,” Journal of Food Engineering, 109 (4) 752—761.
Publisher – Google Scholar
8. Biswal, D. R. and Singh, R. P. (2004) “High performance flocculating agents and viscosifiers based on hydrolysed polyacrylamide grafted amylopectin and polyacrylamide grafted carbox-ymethyl cellulose,” Carbohydrate Polymers, 57 (4) 379-387.
9. Kim, S. K. and Mendis, E. (2006)” Bioactive compounds from marine processing byproducts: A review,” Food Research International, 39 (4) 383-393.
Publisher – Google Scholar
10. Kim, S. K. and Rajapakse, N. (2005)” Enzymatic production and biological activities of chi-tosan oligosaccharides (COS): A review,” Carbohydrate Polymers, 62 (4) 357-368.
Publisher – Google Scholar
11. Shahidi, F. and Abuzaytoun, R. (2005)”Chitin, chitosan, and coproducts: chemistry, produc-tion, applications, and health effects,” Advances in Food and Nutrition Research, 49, 93-135.
Publisher – Google Scholar
12. Venkatesan, J. and Kim, S. K. (2010)” Chitosan composites for bone tissue engineering -An overview,” Marine Drugs, 8 (8) 2252-2266.
Publisher – Google Scholar
13. Venkatesan, J, Pangestuti, R, Qian, Z. J, Ryu, B. and Kim, S. K. (2010)”Biocompatibility and alkaline phosphatase activity of phosphorylated chitooligosaccharides on the osteosarcoma MG63 cell line,” Journal of Functional Biomaterials, 1(1) 3-13.
Publisher – Google Scholar
14. Meyer, H., Butte, W. and Schlaak, M. (2000)”Chitosan in wastewater treatment”. Advances in Chitin Science, 4, ( ) 153-158.
15. Sudha. P. N. (2010) Chitin/chitosan and derivatives for wastewater treatment, Chitin, Chitosan, Oligosaccharides and Their Derivatives. Se-Kwon Kim (ed), CRC press. Chapter 39, 561-576.
16. Caner, H, Hasipoglu, H, Yilmaaz O. and Yilmaaz, E. (1998) “Graft copolymerization of 4-vinylpyridine onto chitosan 1. by ceric ion initiation,” European Polymer Journal, 34 ( ) 493-497.
Google Scholar
17. Pedram, M. Y. and Retuert, J. (1997) “Homogeneous Grafting Reaction of Vinyl Pyrrolidone onto Chitosan,” Journal of Applied Polymer Science, 63 (10)1321-1326.
Publisher – Google Scholar
18. Shantha, K. L, Bala, U. and Rao, K. P. (1995) “Tailor-made chitosans for drug delivery,” European Polymer Journal, 31(4) 377-382.
Publisher – Google Scholar
19. Sun, T. Xie W. and Xu, P. (2004) “Superoxide anion scavenging activity of graft chitosan de-rivatives,” Carbohydrate Polymers, 58 (4) 379-382.
Publisher – Google Scholar
20. Srivastava, A, Tripathy, J, Mishra M. M. and Behari, K. (2007) “Modification of guar gum through grafting of 4-vinyl pyridine using potassium peroxymonosulphate/ ascorbic acid redox pair,” Journal of Applied Polymer Science, 106 (2) 1353-1358.
Publisher – Google Scholar
21. Tripathy, J, Mishra, D. K, Srivastava, A, Mishra M. M. and Behari, K. (2008) “Synthesis of par-tially carboxymethylated guar gum-g-4-vinyl pyridine and study of its water swelling, metal ion sorption and flocculation behaviour,” Carbohydrate Polymer, 72 (3) 462-472.
Publisher – Google Scholar
22. Joshi, J. M. and Sinha, V. K. (2007) Ceric ammonium nitrate induced grafting of polyacrylamide onto carboxymethyl chitosan,” Carbohydrate Polymers, 67 (3) 427—435.
Publisher – Google Scholar
23. Kulkarni, A. G, Mathur, R. M. and Tandon, R. (2006) “Some chemical aspects of color removal from effluents of paper industry,” Journal of Indian Pulp Paper Technical Association, 18 , 55—61.
24. Chen, X., G., Park, H., J., 2003. Chemical characteristics of O-carboxymethyl chitosans related to the preparation conditions,” Carbohydrate Polymers, 53(4) 355-359.
Publisher – Google Scholar
25. Manu, B. (2007) “Physico-chemical treatment of indigo dye wastewater,” Coloration Tech-nology, 123 (3) 197—202.
26. Ranjbar-Mohammadi, M, Aramia, M, Bahramia, H, Mazaheri F. and Mahmoodi, N. M. (2010) “Grafting of chitosan as a biopolymer on to wool fabric using anhydride bridge and its antibacterial property,” Colloids and Surfaces B: Biointerfaces, 76 (2) 397—403.
Publisher – Google Scholar
27. James, A. E. and Nasser, M. S. (2006) “The effect of polyacrylamide charge density and mo-lecular weight on the flocculation and sedimentation behaviour of kaolinite suspensions,” Se-paration and Purification Technology, 52(2) 241—252.
Publisher – Google Scholar
28. Singh, R. P. (1990) Encyclopedia of Fluid Mechanics, Gulf Publishing, Houston, Vol. 9, Chapter 14, p.p. 425—480.
29. Singh, R. P. and Tripathy, T. (2000) “High performance flocculating agent based on partially hydrolysed sodium alginate—g—polyacrylamide,” European Polymer Journal, 36 (7) 1471- 1478.
Publisher – Google Scholar
30. Srivastava, A, Mishra D. K, Tripathy, J, Behari, K. and Mishra, M. M. (2008) “Graft copolymer (chitosan-g-N-vinyl formamide): Synthesis and study of its properties like swelling, metal ion uptake and flocculation,” Carbohydrate Polymers, 74 (3) 632—639.
Publisher – Google Scholar
31. Radeva, T. (2001) Physical Chemistry of Polyelectrolytes, Marcel Dekker Inc., New York, USA.
32. Rey, F. A. and Varsanik, R. C. (1986) Application and function of synthetic polymeric floccu-lents in wastewater treatment, Water Soluble Polymers: Beauty with Performance, Glass, J. E. (ed), pp. 113—143, ACS Advances in Chemistry Series No. 213, American Chemical Society, Washington, D.C.
33. Hjerde, R. J. N, Vårum, K. M, Grasdalen, H, Tokura, S. and Smidsrød, O. (1997) “Chemical composition of O-(carboxymethyl)-chitins in relation to lysozyme degradation rates,” Carbohy-drate Polymers, 34 (3) 131-139.
Google Scholar
34. Windebank, A. J, Kempen, D. H, Lewis, J. L, Lu, L. C, Yaszemski, M. J, Simha, N. X. and Wang, S. F. (2008) ”Photo-cross-linked hybrid polymer networks consisting of poly (propylene fumarate) and poly(caprolactone fumarate): controlled physical properties and regulated bone and nerve cell responses,” Biomacromolecules, 9 (4) 1229—1241.
Publisher – Google Scholar
35. Wirsen, A, Albertson, A. C. and Qu, X. (1999) “Structural change and swelling mechanism of pH-sensitive hydrogel based on chitosan and D, L- lactic acid,” Journal of Applied Polymer Science, 74, 3193ï€3202.
Google Scholar
36. Yumin, D., Houbin, L., Yongmei, X., Huaiyu, Z., Kennedy, J., F. (2004) “Interactions of cationized chitosan with components in a chemical pulp suspension,” Carbohydrate Polymers, 58 (2) 205-214.
Publisher – Google Scholar
37. Zhu, A, Li, L, Chan-Park, M. B. and Dai, S. (2005) “The aggregation behaviour of o-carboxymethyl chitosan in dilute aqueous solution,” Colloids and Surfaces B: Biointerfaces, 43 (3-4) 143-149.
Publisher – Google Scholar